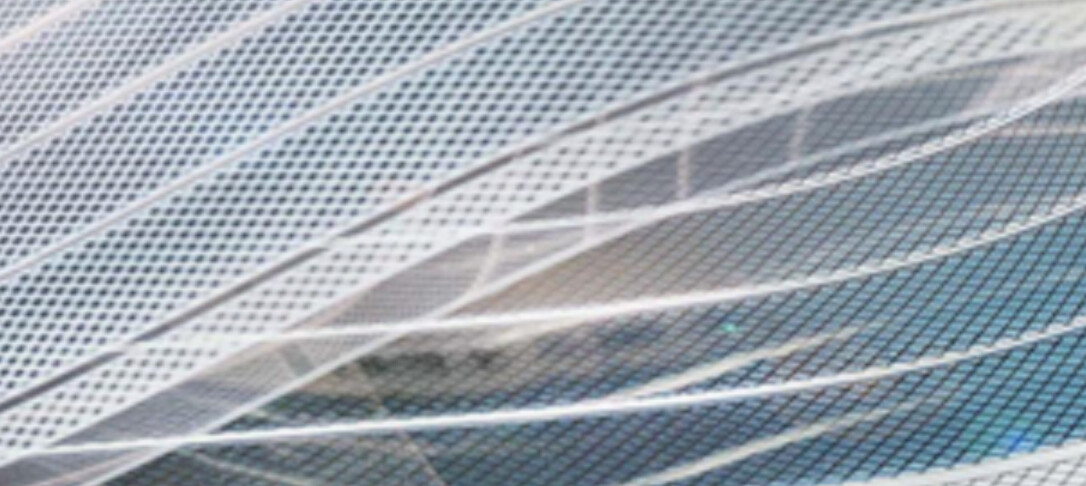
The symposium aims to bring frontier researchers together to discuss the photoconversion in organic and hybrid semiconductors, their fundamental science and applications towards sustainable energy. The symposium is co-sponsored by Imperial’s Centre for Processable Electronics and the UKRI project ATIP. We are delighted to invite you to join us for this exciting discussion.
Programme:
0900-0930 Welcome tea/coffee
0930-0940 Welcome remarks (Prof Ji-Seon Kim)
Chair: Prof Ji-Seon Kim
0940-1020 Dr Johannes Bendhun, Technische Universitat Dresden: Single-component vacuum-deposited organic photodetector for high-performance sensing
1020-1100 Prof Koen Vandewal, University of Hasselt: New device architectures and performance limitations of organic infrared sensors
1100-1120 Tea/coffee break
Chair: Dr Julie Euvrard
1120-1200 Prof Annamaria Petrozza, Italian Institute of Technology: Defects activity in metal halide perovskites
1200-1240 Prof Sam Stranks, University of Cambridge: Optical spectroscopy to drive optoelectronic device development
1240-1340 Lunch
Chair: Dr Nicola Gasparini
1340-1420 Prof Sabine Ludwigs, University of Stuttgart: Adaptive semiconducting polymer films for optoelectronics and switchable devices
1420-1500 Prof Jenny Nelson, Imperial College London: Talk title TBC
1500-1520 Tea/coffee break
Chair: Dr Salva Eslava
1520-1600 Prof Erwin Reisner, University of Cambridge: Integrated solar chemistry devices for sustainable synthesis
1600-1640 Prof James Durrant, Imperial College London: Charge carrier kinetics in organic solar cells and photocatalysts
1650-1750 Panel discussion moderated by Dr Alex Ganose, Dr Katie Stewart and Dr Julianna Panidi
____________________________________
ABSTRACTS AND BIOS
Single-component Vacuum-deposited Organic Photodetector for High-performance Sensing
Dr Johannes Benduhn, Dresden Integrated Center for Applied Physics and Photonic Materials (IAPP) and Institute of Applied Physics (IAP) Technische Universität Dresden
Organic semiconductors have proven to be a versatile technology platform and are compatible with high-scale mass production. Vacuum-deposited organic light-emitting diodes (OLEDs) dominate mobile display applications and are superior due to their mechanical flexibility, high contrast, low-cost, and form-free production. Recently, organic photodetectors have attracted much interest since they are directly compatible with the OLED display technology and have excellent potential to complement it. Furthermore, their physical properties, such as semitransparency, flexibility, narrowband detection, and, most importantly, high detectivity, offer new perspectives for optoelectronic sensing and will stimulate new consumer electronics applications.
Typically, organic solar cells and photodetectors are comprised of an electron donating and accepting material to facilitate efficient charge carrier generation. This approach has proven successful in achieving high-performance devices but has several drawbacks. For example, creating the proper donor-acceptor microstructure is critical to achieve the desired performance. However, this is challenging when it comes to upscaling and is considered one of the significant degradation pathways regarding the stability of these devices. Therefore, we investigate a vacuum-deposited oligothiophene A-D-A molecule in a single-component device and find that it can generate free charge carriers with an external quantum efficiency of 20% already at zero bias. Optimizing the device structure, we achieve specific detectivities of 1E13 Jones (based on noise measurements), high speed (f-3dB = 330 kHz), and linear dynamic range (190 dB). To unveil the charge carrier generation within this material system, we employ ultrafast transient absorption spectroscopy and quantum chemical calculations and find that free charge carriers are already formed at time scales below one picosecond. Exhibiting this excellent performance and using the simple device structure, such single-component devices are perfect candidates for application.
BIO
Johannes Benduhn studied Physics at the University of Greifswald (Germany), University of Wrocław (Poland), and Technische Universität Dresden (Germany), where he obtained his Master of Science (2015) and Doctorate degree (2019) under the supervision of Prof. Koen Vandewal. Afterward, he joined the start-up Senorics GmbH, which expedites mobile spectroscopy solutions based on organic photodetectors. Currently, Johannes Benduhn is leading the research group “Organic Sensors and Solar Cells” at Technische Universität Dresden at the professorship of Prof. Karl Leo. His main research interests range from studying fundamental processes in organic semiconductors to improving optoelectronic devices by employing new materials and exploring new device architectures.
Adaptive Semiconducting Polymer Films for Optoelectronics and Switchable Devices
Prof. Dr. Sabine Ludwigs, IPOC – Functional Polymers, Institute of Polymer Chemistry, University of Stuttgart
The presentation will give an overview over recent studies in my group about design, processing and characterization of polymeric conductors for opto-electronic and switchable technologies including organic solar cells, electrically switchable surfaces and actuators for soft robotics.
Electrochemistry is shown as versatile tool to control the doping level of semiconducting polymer films. Data on tunable UV-vis-NIR absorption properties and electrical conductivities are presented for p-type and n-type semiconducting polymer materials.1 Both, in-situ as well as ex-situ electrochemical techniques are discussed and correlations to the morphology of the films are established. For energy level calculation the method of spectral onset determination from in-situ spectroelectrochemistry measurements has been established. This proves particularly useful for blend films in fully organic solar cells.2
Beyond opto-electronic applications, electrochemical switching of the mixed conductor poly(ethylenedioxythiophene):poly(styrenesulfonate) (PEDOT:PSS) results in different degrees of volume swelling in organic and in aqueous electrolytes as characterized by in-situ spectroscopic ellipsometry.3 The humidity dependence of the PSS polyelectrolyte phase further allows to induce volume changes by water uptake. The bending behavior of “intelligent” humidity-triggered4 and electrochemically-triggered bilayer actuators is highlighted for soft robotic applications.
1 D. Neusser, C. Malacrida, M. Kern, Y. Gross, J. van Slageren, S. Ludwigs, High Conductivities of Disordered P3HT films by an Electrochemical Doping Strategy, Chem. Mater. 2020, 32, 6003.
2 D. Neusser, B. Sun, W.L. Tan, L. Thomsen, T. Schultz, L. Perdigon-Toro, N. Koch, S. Shoaee, C.R. McNeill, D. Neher, S. Ludwigs, Spectroelectrochemically Determined Energy Levels of PM6:Y6 Blends and their Relevance to Solar Cell Performance, J. Mater. Chem. C 2022, 10, 11565.
3 C. Dingler, R. Walter, B. Gompf, S. Ludwigs, In-situ Monitoring of Optical Constants, Conductivity and Swelling of PEDOT:PSS from Doped to the Fully Neutral State, Macromolecules 2022, 55, 1600.
4 C. Dingler, H. Müller, M. Wieland, D. Fauser, H. Steeb, S. Ludwigs Understanding Mechanical Behavior to Curvature Prediction of Humidity-Triggered Bilayer Actuators, Adv. Mater. 2021, 202007982.
BIO
Sabine Ludwigs has been a full professor of polymer chemistry since 2010 and holds the Chair of Structure and Properties of Polymeric Materials at the Institute of Polymer Chemistry of the University of Stuttgart. After diploma studies of chemistry and a dissertation in physical chemistry in 2004 at the University of Bayreuth, she worked as a postdoc at the Cavendish Laboratory of the University of Cambridge for two years. From 2006 until 2010 she set up her own independent group at the University of Freiburg, e.g. by funding via an Emmy Noether grant of the German Science Foundation. Since 2023 she has been co-speaker of a graduate training group on Mixed Ionic-Electronic Transport of Heidelberg University and the University of Stuttgart (RTG 2948).
Defects Activity in Metal Halide Perovskites
1 Center for Nano Science and Technology @Polimi, Istituto Italiano di Tecnologia; Milan, Italy
E–mail: annamaria.petrozza@iit.it
Annamaria Petrozza leads the “Advanced Materials for Optoelectronics” research group and she is the Director of the Center for Nano Science and Technology of the Italian Institute of Technology. She received her PhD in Physics in 2009 from the University of Cambridge (UK) with a thesis on the study of optoelectronic processes at organic and hybrid semiconductors interfaces under the supervision of Prof. J.S. Kim and Prof Sir R.H. Friend. From 2008 to 2010 she worked as a staff scientist at the Sharp Laboratories of Europe, Ltd (Oxford, UK) on the development of new market competitive solar cell technologies.
She got the “Innovators Under 35 Italy 2014” award by the MIT Technology Review for her pioneering work on perovskites. She has been selected among the “Emerging Investigators 2017” by the Royal Society of Chemistry. In 2022 she was awarded “The Innovation in Materials Characterization Award” by the Materials Research Society which honors an outstanding advance in materials characterization. Since 2023 she has been a Fellow of the Royal Society of Chemistry. She serves as senior editor at “ACS Energy Letters” (American Chemical Society).
Integrated Solar Chemistry Devices for Sustainable Synthesis
Erwin Reisner
Yusuf Hamied Department of Chemistry, University of Cambridge, Lensfield Road, Cambridge CB2 1EW, UK
Solar panels are well established to produce electricity as photovoltaic cells and are already in development to photo-catalyse overall water splitting to produce green hydrogen as artificial leaves or photocatalyst sheets.1,2 This presentation will introduce solar chemistry panels as an emerging technology to enable sunlight-powered circular carbon chemistry (Figure 1). Our progress in designing and constructing prototype solar devices for the direct conversion of carbon dioxide as well as the valorisation of biomass and plastic waste streams into renewable fuels and higher-value chemicals will be presented.
Specifically, a standalone artificial leaf based on an integrated lead halide perovskite-BiVO4 tandem light absorber architecture with immobilised molecular catalysts has been created for solar CO2 reduction to produce syngas (CO and H2) fuel coupled to oxygen (O2) evolution from water oxidation.3 Further manufacturing advances have enabled the reduction of material requirements to fabricate light weight devices that float on water, thereby enabling applications on open water sources instead of requiring land for installation.4 The versatile tandem design also allows for the integration of biocatalysts and thus the assembly of semi-artificial photosynthetic devices, demonstrating selective and bias-free conversion of CO2-to-formate using immobilised enzymes.5 Recent progress in catalyst-development has allowed us to show carbon-carbon bond formation and the direct production of ethanol and propanol directly from CO2, establishing artificial photosynthesis to produce liquid multicarbon fuels.6 The encapsulated perovskite photoelectrodes also provide a platform for the assembly of wireless solar devices for the valorisation of biomass and plastic waste through solar reforming (instead of oxidising water), 7,8 as well as the coupling to CO2-to-fuel conversion,8 including atmospheric CO2 through integrated direct air capture.9
An alternative solar carbon capture and utilisation technology is based on co-deposited semiconductor powders on a conducting substrate.2 Modification of these immobilised powders with a molecular catalyst provides us with a photocatalyst sheet that can cleanly produce formate from aqueous CO2 while co-producing O2.10 CO2-fixing bacteria grown on such tandem photocatalyst sheets enable the production of multicarbon products through clean CO2-to-acetate conversion.11 The deposition of a single semiconductor material on glass allows sunlight-driven plastic and biomass waste upcycling to organic products coupled to hydrogen evolution or CO2-to-fuel conversion, thereby allowing for simultaneous waste remediation and fuel production.12,13
The concept and prospect of integrated solar chemistry panels for artificial photosynthesis and solar reforming,14,15 strategies to improve light management in such devices16 and their relevance to secure and harness sustainable energy supplies in a circular economy will be discussed.
Figure 1. Solar panel technologies for integrated solar-to-chemical conversion. Artificial leaves and photocatalyst sheets use sunlight to power artificial photosynthesis using a tandem architecture or waste valorisation through solar reforming using a single light absorber architecture. Figure from ref. 14.
References
[1] Reece et al., Science, 2011, 334, 645–648; [2] Wang et al., Nat. Mater., 2016, 15, 611–615; [3] Andrei et al., Nat. Mater., 2020, 19, 189–194; [4] Andrei et al., Nature, 2022, 608, 518–522; [5] Moore et al., Angew. Chem. Int. Ed., 2021, 60, 26303–26307; [6] Rahaman et al., Nat. Energy, 2023, 8, 629–638; [7] Bhattacharjee et al., Adv. Funct. Mater., 2022, 32, 2109313; [8] Bhattacharjee et al., Nat. Synth., 2023, 2, 182–192; [9] Kar et al., Joule, 2023, 7, 1496–1514; [10] Wang et al., Nat. Energy, 2020, 5, 703–710; [11] Wang et al., Nat. Catal., 2022, 5, 633–641; [12] Uekert et al., Nat. Sustain., 2021, 4, 383–391; [13] Bhattacharjee et al., J. Am. Chem. Soc., 2023, 145, 20355–20364; [14] Andrei et al., Acc. Chem. Res., 2022, 55, 3376–3386; [15] Bhattacharjee et al., Nat. Rev. Chem., 2024, in print (DOI: 10.1038/s41570-023-00567-x); [16] Wang et al., Nat. Energy, 2022, 7, 13–24
BIO
Erwin Reisner is the Professor of Energy and Sustainability in the Department of Chemistry at the University of Cambridge and a Fellow of St. John’s College. He is an expert in renewable energy and sustainable chemistry, in particular the sunlight-powered production of sustainable fuels and platform chemicals. His cross-disciplinary research into solar chemical synthesis technologies focuses on the capture and utilisation of the greenhouse gas carbon dioxide as well as the valorisation of plastics and biomass waste to produce green fuels and chemicals for a circular economy.
Optical Spectroscopy to Drive Optoelectronic Device Development
Sam Stranks
Optical spectroscopy provides a powerful toolset to understand material and behaviour. Here, I will outline recent work where we have adapted and utilised high spatial and time-resolution optical tools to study a range of material systems including halide perovskite solar cells and LEDs, and copper-based photocathodes. I will show results where we use luminescence mapping measurements on full operating perovskite solar cells to extract information about local performance losses, and understand evolution of the solar cells under long-term operation. These measurements are correlated with local structure and chemical mapping measurements to understand the origins of charge carrier trapping, performance losses and instabilities. From a new materials perspective, I will show how transient optical measurements reveal charge transfer between inorganic perovskite backbones and functional 2D spacer molecules, demonstrating that these molecules can be electroactive, thereby opening up new functionalities. I will also show our latest results on high-performance blue and white perovskite LED device developments driven by optical spectroscopy. Finally, I will show results where transient reflection spectroscopy measurements are used to demonstrate that carrier mobility along the [111] orientation of Cu2O photocathodes are an order of magnitude higher than along other orientations, leading to high performing and stable operation of photoelectrochemical devices.
BIO
Sam Stranks is Professor of Optoelectronics in the Department of Chemical Engineering and Biotechnology at the University of Cambridge, and a Fellow of the Royal Society of Chemistry and the Institute of Physics. Sam’s research focuses on the optical and electronic properties of emerging semiconductors including halide perovskites and organic semiconductors for low-cost electronics applications such as photovoltaics, lighting and detectors. He received the 2018 Henry Moseley Award and Medal from the Institute of Physics the 2019 Marlow Award from the Royal Society of Chemistry, the 2021 IEEE Stuart Wenham Award, the 2021 Leverhulme Prize in Physics, the 2021 EES Lectureship and 2024 Blavatnik Finalist Award in Chemical Sciences. Sam is also co-founder of Swift Solar, a startup developing high-performance perovskite PV panels, and Sustain/Ed, a not-for-profit developing education for school-age children around climate change solutions.
New Device Architectures and Performance Limitations of Organic Infrared Sensors
Koen Vandewal
Organic photodetectors (OPDs) with a performance comparable to that of conventional inorganic ones have been demonstrated for the visible regime. However, infrared (IR) photodetection has proven to be challenging and, to date, the true potential of organic semiconductors in the near-infrared spectral range (800–2500 nm) and beyond remains largely unexplored. In this talk, I will introduce new device concepts for organic IR detectors, based on resonant optical cavities and doped photo-active layers. Design rules and optimization strategies will be discussed, yielding broadband, as well as wavelength selective devices with a tunability of the detection wavelength over several hundreds of nanometers, allowing the printing of miniature NIR spectrometers. In a second part of the talk, we explore the performance limitations of organic IR detectors: A relation between open-circuit voltage, dark current, and noise current is demonstrated for OPDs with detection wavelengths beyond 1100 nm. Based on these findings we estimate an upper limit of achievable specific detectivity values for organic photodiodes as a function of their longest IR detection wavelength.
BIO
Prof. Dr. Koen Vandewal obtained his PhD in Physics at Hasselt University in 2009 working on the device physics of organic photovoltaics. After that, he has been working for two years as a Postdoctoral Fellow at Linkoping University in Sweden and another two years at Stanford University in the US. In 2014, he was appointed as endowed professor at the Technische Universitaet (TU) Dresden in Germany. In January 2018, he moved from TU Dresden to Hasselt University leading a research group with the aim to solve fundamental questions in the field of organic, hybrid and molecular electronics with relevance to applications in opto-electronic devices such as organic light emitting diodes, solar cells, energy conversion devices and sensors.
Charge carrier kinetics in organic solar cells and photocatalysts
James R Durrant
There is increasing interest in harnessing sunlight to drive the synthesis of molecular fuels and chemicals, including in particular water photolysis to yield molecular oxygen and hydrogen. This can be achieved either through the coupling of photovoltaic cells and electrolysis, or through direct sunlight conversion by photoelectrodes or photocatalysts, the latter being the focus of this talk. In solar conversion, there is often a critical kinetic mismatch between the lifetimes of initially generated photoexcited states and the timescales of charge extraction / catalysis. I will start my talk by discussing this charge carrier lifetime challenge in photoelectrochemical and photocatalytic systems and how this challenge is greater than in organic and perovskite solar cells. I will briefly exemplify this with examples from organic and perovskite solar cells, drawing up our work as part of the ATIP project. I will then go on to discuss in more detail some of our recent studies employing transient optical spectroscopies measuring charge carrier dynamics in photoelectrodes and photocatalysts and how these impact upon the efficiency of harnessing sunlight, focusing primarily on conjugated organic materials. I will briefly introduce this challenge with examples from metal oxide photoelectrodes and photocatalysts. I will then go on to discuss carbon nitride and organic polymer photocatalysts, and the impact of charge trapping and heterojunctions in extending the lifetime of charge carriers. I will conclude by directly contrasting charge carrier dynamics in organic bulk heterojunction films versus particles in water, and highlight the major impact of water exposure on these charge carrier dynamics and energetics.
BIO
James Durrant is Professor of Photochemistry in the Department of Chemistry, Imperial College London and Sêr Cymru Solar Professor, College of Engineering, University of Swansea. His research focuses on the use of transient laser spectroscopy and optoelectronic technniques to investigate the function of new materials for sustainable energy conversion, including materials for artificial photosynthesis, solar cells and electrolysis. More widely, as part of the SPECIFIC IKC, he leads the EPSRC programme grant ATIP, and at Imperial leads its Centre for Processable Electronics (the CPE).