This one-day meeting brings together specialists in the areas of drop impact onto liquid and solid surfaces. The event has attracted very strong participation from institutions across the United Kingdom and mainland Europe. Speakers from varied backgrounds developing analytical, computational as well as experimental methods will present their recent work on this fascinating subject.
Invited speakers:
- Alfonso Castrejon-Pita, University of Oxford, United Kingdom
- Jens Eggers, University of Bristol, United Kingdom
- Jose Manuel Gordillo, Universidad de Sevilla, Spain
- Peter Hicks, University of Aberdeen, United Kingdom
- Alexander Korobkin, University of East Anglia, United Kingdom
- Pierre-Yves Lagree, UPMC Universite Paris 06, France
- Jie Li, University of Cambridge, United Kingdom
- Guillaume Riboux, Universidad de Sevilla, Spain
- Frank Smith, University College London, United Kingdom
- James Sprittles, University of Warwick, United Kingdom
- Robert Style, ETH Zurich, Switzerland
- Stephen Wilson, University of Strathclyde, United Kingdom
- Zhihua Xie, Imperial College London, United Kingdom
The event is generously supported by the Centre for Computational Methods in Science and Engineering of Imperial College London.
Register here
Further information
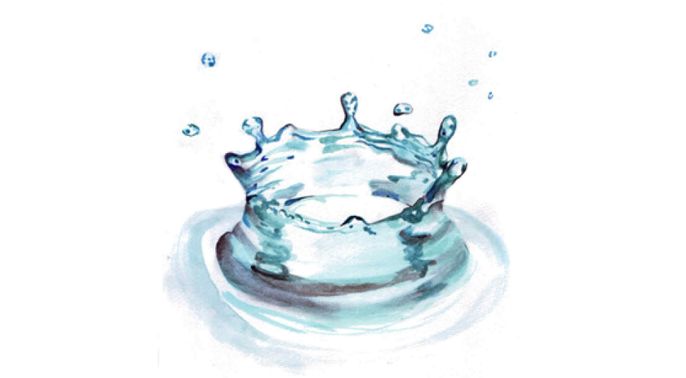
Scientific programme
Contributions consist in 25-minute presentations distributed in four sessions throughout the day. View the Drop Impact meeting schedule.
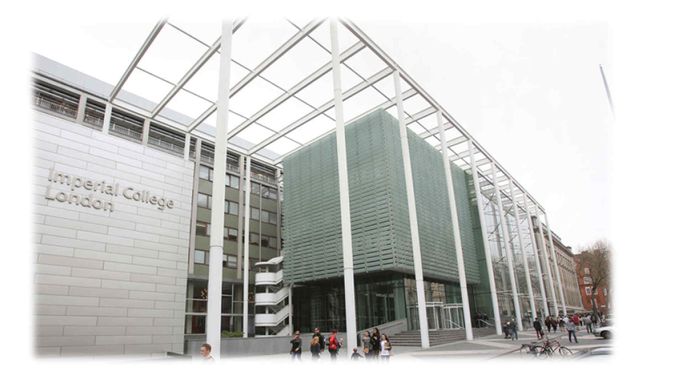
How to get here
The meeting is held in Lecture Theatre 3 on the third floor of the Imperial College Business School. The building is most conveniently accessed via Exhibition Road, coming from the South Kensington Underground Station.
Abstracts
- Alfonso Castrejon-Pita, University of Oxford, United Kingdom
- Jens Eggers, University of Bristol, United Kingdom
- Jose Manuel Gordillo, Universidad de Sevilla, Spain
- Peter Hicks, University of Aberdeen, United Kingdom
- Alexander Korobkin, University of East Anglia, United Kingdom
- Pierre-Yves Lagrée, UPMC Université Paris 06, France
- Jie Li, University of Cambridge, United Kingdom
- Guillaume Riboux, Universidad de Sevilla, Spain
- Frank Smith, University College London, United Kingdom
- James Sprittles, University of Warwick, United Kingdom
- Robert Style, ETH Zurich, Switzerland
- Stephen Wilson, University of Strathclyde, United Kingdom
- Zhihua Xie, Imperial College London, United Kingdom
Drop deposition onto a variety of surfaces including solids, liquid pools, powders and other complex materials is a very active research topic with a wide range of applications, ranging from understanding how rainfall penetrates surfaces, to pesticide application, inkjet printing, fuel combustion, forensic science and spray coating. In this chat I will present a few recent and current experimental studies in this topic, ranging from drop deposition onto other drops and liquid (static and moving) pools to drop impact onto soft solids.
Drop dynamics after impact on a solid wall
I report on the impact of a fluid drop onto a planar solid surface at high speed so that at impact,
kinetic energy dominates over surface energy and inertia dominates over viscous effects. As the
drop spreads, it deforms into a thin film, whose thickness is limited by the growth of a viscous
boundary layer near the solid wall. Owing to surface tension, the edge of the film retracts relative
to the flow in the film and fluid collects into a toroidal rim bounding the film. Using mass and
momentum conservation, we construct a model for the radius of the deposit as a function of time.
At each stage, we perform detailed comparisons between theory and numerical simulations of
the Navier–Stokes equation.
Drop splashing at smooth dry surfaces
When a drop impacts a smooth, dry surface at a velocity above the so-called critical speed for drop splashing, the initial liquid volume loses its integrity, fragmenting into tiny droplets that are violently ejected radially outwards. Making use of experiments, potential flow and lubrication theories and of numerical simulations, we first develop a model to predict the critical velocity for splashing. We find that dewetting is a necessary but not sufficient condition for splashing. Splashing only occurs when the drop velocity is such that, the much faster and thinner liquid sheet which is expelled in the direction tangent to the solid as a consequence of the impact, is accelerated vertically up to velocities larger than those caused by the capillary retraction of the sheet. The vertical accelerations are imparted to the edge of the spreading liquid sheet by the aerodynamic lift force exerted by the surrounding gas on the edge of the radially expanding lamella. The lift force per unit length results as the addition of: i) the classical term used in aerodynamics (which depends on the product of gas density, the squared relative velocity and the thickness of the lamella) plus ii) a term that depends on gas viscosity as the product of four terms: gas viscosity, the velocity of the liquid sheet edge relative to that of the ambient gas, a constant which is a function of the angle the advancing lamella forms with the substrate and a logarithm which incorporates the ratio between the thickness of the lamella and the mean free path of molecules. The contribution of the logarithm, and as a result, of the mean free path, is essential since, otherwise, these “viscous lift forces” would tend to infinite. The wedge angle is not the static contact angle with the substrate, but it is the angle the advancing liquid front forms with the solid substrate. Our splash criterion can be summarized as follows: if the vertical velocities imparted to the edge of the sheet by the aerodynamic lift forces are larger than the radial velocity at which the edge of the sheet grows by capillary retraction, the edge of the sheet “takes off” and the toroidal rim bordering the sheet is prone to develop Savart-Plateau-Rayleigh capillary instabilities. If the growth time of capillary instabilities is sufficiently small when compared with the time of growth of the toroidal rim, the edge of the lamella breaks into very tiny drops, of sizes comparable to that of the thickness of the rim.
For impact velocities larger than the critical splash velocity and making use of a one-dimensional approximation describing the flow in the ejected liquid sheet and of balances of mass and momentum at the border of the sheet, we predict the mean sizes and velocities of the ejected drops. The predictions of the model are in good agreement with experiments.
Gas-cushioned droplet impacts with porous substrates
Gas cushioning in idealized two-dimensional droplet impacts with an isotropic porous layer located above an impermeable surface is investigated. Distinguished limits for shallow, finite and deep porous layers will be described. In each case the minimum effective substrate permeability required to create a leading-order contribution to gas cushioning is identified. In low effective permeability regimes, the gas pressure build-up between the droplet and the substrate causes the droplet free-surface to decelerate before touchdown, trapping a pocket of gas between the droplet free surface and the substrate. Experimentally validated scaling laws exist for the initial dimensions of the trapped gas pocket in an impact with an impermeable substrate. Here the functional dependence of the scaling law “pre-factor” upon the substrate permeability and the thickness of the porous layer will be illustrated, showing that the initial dimensions of the trapped gas pocket decreases with increases in both the effective permeability and porous layer thickness. With a permeable substrate, air can escape from the path of the oncoming droplet through the substrate and hence the effectiveness of gas cushioning is reduced. In cases of high effective permeability, while the droplet free-surface decelerates, the pressure build-up may be insufficient to produce a trapped a gas pocket, with touchdown occurring immediately below the droplet centre.
Water entry and exit by simplified models and CFD
The two-dimensional problem of a symmetric wedge entering water and exiting from it thereafter at a
time-dependent speed is considered. The pressure distribution along the wetted part of the wedge and
the total hydrodynamic force acting on the wedge are calculated by three simplified models: the Original
Wagner Model (OWM) of water entry and the Linearized Model of water exit (LME), the Modified Logvinovich
Model (MLM) of entry and exit, and the Generalized Wagner Model (GWM) of entry and exit. Computational Fluid Dynamics (CFD) simulations are also used to generate reference results for the
development and elaboration of the water exit models with large displacements. A custom solver (CFD1)
built on the open-source finite-volume CFD library OpenFOAM was originally used to numerically solve
the Navier-Stokes equations governing the air-water flow. To start with the exit models, one needs to ensure
that the entry stage is well predicted by both CFD and simplified models. However, the hydrodynamic forces
and the pressure distributions predicted by the GWM from Khabakhpasheva et al. (2014) and by the MLM from
Korobkin (2004) were found to be rather different from those by the CFD during the entry stage. To examine
why the force predictions are so different, only vertical motions of a symmetric wedge with the deadrise angle
of 45 degrees were considered in detail, and special attention was paid to the end of the entry stage, where
the wedge speed is small. The accuracy of the CFD results was confirmed by running each case of the wedge motions twice by the
OpenFOAM solvers developed by Piro and Maki (2013, CFD1) and by Seng et al. (2014, CFD2). It was found that
both OpenFOAM solvers provide close results. The obtained results demonstrate that the gravity provides an
important contribution to the force at the end of the entry stage. The gravity effects were included in the
symplified models of water entry/exit.
Drop impact on a solid surface: short time self-similarity
Macroscopic model for head-on binary droplet collisions in a gaseous medium
In this work, coalescence-bouncing transitions of head-on binary droplet collisions are predicted by a novel macroscopic model based entirely on fundamental laws of physics. By making use of the lubrication theory of Zhang and Law [Phys. Fluids 23, 042102 (2011)], we have modified the Navier-Stokes equations to accurately account for the rarefied nature of the interdroplet gas film. Through the disjoint pressure model, we have incorporated the intermolecular Van der Waals forces. Our model does not use any adjustable (empirical) parameters. It therefore encompasses an extreme range of length scales (more than 5 orders of magnitude): from those of the external flow in excess of the droplet size (a few hundred micros) to the effective range of the Van der Waals force around 10 nm. A state of the art moving adaptive mesh method, capable of resolving all the relevant length scales, has been employed. Our numerical simulations are able to capture the coalescence-bouncing and bouncing-coalescence transitions that are observed as the collision intensity increases. The predicted transition Weber numbers for tetradecane and water droplet collisions at different pressures show remarkably good agreement with published experimental values. Our study also sheds new light on the roles of gas density, droplet size and mean free path in the rupture of the gas film.
Drop splashing on smooth heated solid substrates
It is our purpose in this talk to predict the critical Weber number for splashing when a drop impacts onto a smooth heated solid surface, namely, the critical impact velocity for which the impacting drop disintegrates into tiny droplets ejected tangentially to the solid wall. For this purpose, we will distinguish two different cases: firstly, we consider the case in which the solid temperature is below the Leidenfrost temperature TL and incorporate the dependence of the physical properties with temperature in the theory of Riboux & Gordillo, PRL, 113, 2014 to determine the critical velocity for the splash transition. Secondly, we consider the case of solid substrate temperatures above TL , for which a vapour layer prevents the drop from touching the solid. As a consequence of this, the splashing morphology differs from the one below the Leidenfrost temperature because, thanks to the presence of the gas layer, the shear stresses acting on the liquid can be neglected. Taking this fact into account, we applied our model to compute the instant the liquid lamella is ejected tangentially to the substrate. Then, the time evolution of the liquid velocity as well as the height of the liquid layer at the junction of the drop with the liquid lamella, are computed using Boundary Integral Simulations. Whereas the liquid velocity agrees for all instants of time with our prediction, the numerically calculated height of the lamella is in agreement with the linearized potential flow theoretical prediction only for short times after impact. The numerical results are then used as boundary conditions for the one-dimensional mass and momentum equations governing the dynamics of the toroidal rim limiting the edge of the lamella - Riboux & Gordillo, JFM, 772, 2015 -. Our predictions for both the maximum spreading radius and for the critical Weber number for splashing, are in good agreement with experimental observations, see figure below.
Distortion and impact of a droplet moving through surrounding fluid
The evolution of such a moving droplet is often captured accurately by direct numerical simulation. On the other hand accuracy tends to be limited when the ratio of the density of the droplet to that of the surrounding fluid becomes small or large and similarly when the viscosity ratio becomes small or large. A complementary approach may shed helpful light then. The present approach takes advantage of extreme ratios through use of matched asymptotic expansions. The scalings accommodate the interfacial conditions holding at the unknown droplet surface as well as other effects. The analysis shows that (e.g.) a lighter less viscous surrounding fluid can move as if past a given shape of the relatively heavy more viscous droplet: there is only slight distortion then. This first stage of evolution is followed later by a stage of full distortion of the droplet. The present approach holds true at any Reynolds number, Weber number or Froude number and has wide application in principle. Subsequent impact and phase change will also be discussed.
Kinetic Effects in Dynamic Wetting
The maximum speed at which a liquid can wet a solid is limited by the need to displace gas lubrication films in front of the moving contact line. The characteristic height of these films is often comparable to the mean free path in the gas so that hydrodynamic models do not adequately describe the flow physics. In this talk, I will develop a model which incorporates kinetic effects in the gas, via the Boltzmann equation, and can predict experimentally-observed increases in the maximum speed of wetting when (a) the ambient pressure is reduced or (b) the meniscus is confined.
Making a splash on soft surfaces
Splashing is actually quite hard to avoid. Current techniques for eliminating splashing include reducing the air pressure, tilting a surface, or moving a surface away from an oncoming droplet at speed. However, these are rather impractical for giving all-round splash protection. However, I’ll demonstrate how simply coating a surface with a thin layer of a soft solid does a remarkably good job. A mixture of numerics and simple theory reveals the mechanism underlying these experimental results.
The Lifetimes of Evaporating Droplets
Evaporating droplets occur in many different situations including coating flows, printing, patterning as well as in nature, and have been the subject of intensive study in recent years. However, one aspect which has received relatively little attention is now the mode in which it evaporates effects the lifetime of an evaporating droplet depends on the mode. We use a combination of analytical and simple numerical techniques to bring some unexpected new insights into this practically important problem.
[1] Dunn, G.J., Wilson, S.K., Duffy, B.R., David, S., Sefiane, K. The strong influence of substrate conductivity on droplet evaporation, J. Fluid Mech. 623 329-351 (2009)
[2] Stauber, J.M., Wilson, S.K., Duffy, B.R., Sefiane, K. On the lifetimes of evaporating droplets, J. Fluid Mech. 744 R2 (2014)
[3] Stauber, J.M., Wilson, S.K., Duffy, B.R., Sefiane, K. Evaporation of droplets on strongly hydrophobic substrates, Langmuir 31 (12) 3653-3660 (2015)
[4] Stauber, J.M., Wilson, S.K., Duffy, B.R., Sefiane, K. On the lifetimes of evaporating droplets with related initial and receding contact angles, Phys. Fluids 27 (12) 122101 (2015)
Three-dimensional numerical simulations of two- and three-phase droplet impact
Multiphase flows where two or more fluids have interfacial surfaces are often found in industrial engineering applications, including bubbles and droplets. The objective of this study is to investigate the fluid dynamics of three-dimensional two- and three-phase droplet impact problems, such as two-phase normal or oblique droplet impact on stationary or moving liquid, three-phase droplet impacting upon a gas-liquid interface and bubble rising through a liquid-liquid interface. An adaptive unstructured mesh modelling framework is employed here to study two- and three-phase flow problems, which can modify and adapt unstructured meshes to better represent the underlying physics of multiphase problems and reduce computational effort without sacrificing accuracy. The numerical framework consists of a mixed control volume and finite element formulation, a ‘volume of fluid’ type method for the interface capturing based on a compressive control volume advection method and second-order finite element methods, and a force-balanced algorithm for the surface tension implementation. Numerical examples of some benchmark tests and the dynamics of two- and three-phase flows are presented to demonstrate the capability of this method.
Organising committee:
- Radu Cimpeanu, Imperial College London, United Kingdom
- Matthew Moore, Imperial College London, United Kingdom
- Demetrios Papageorgiou, Imperial College London, United Kingdom
The creative artwork on drop impact for this meeting was designed by Anca Pora Illustration.
Register here
For registration please fill in this form.
Meeting details
Date: Friday, 11th November
Venue: ICBS 300 LT3