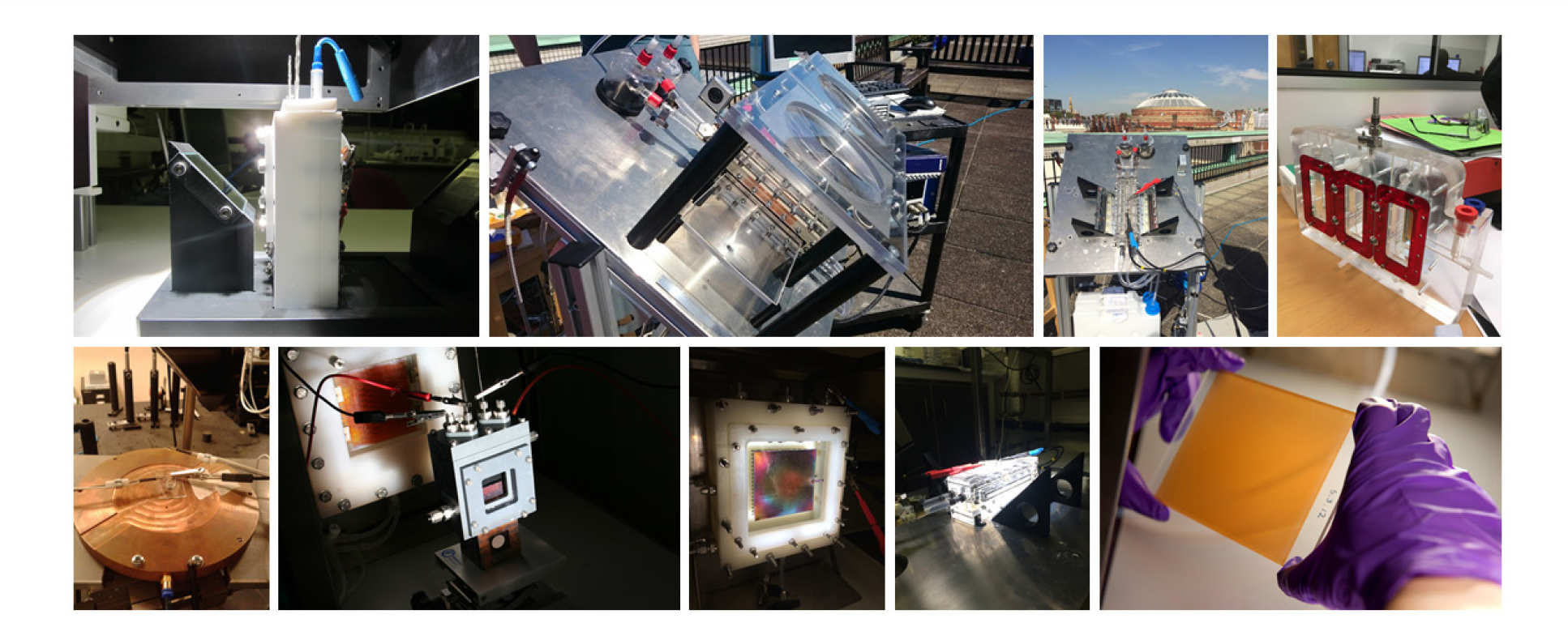
Our goal is to design and build scalable prototype devices for spontaneous splitting of water into hydrogen and oxygen upon illumination by solar photons. This is one of the potential routes to sustainable, carbon-free energy.
The principal considerations in the design of such systems are materials and reactor structure; in our group we research both in parallel. We synthesize photoabsorbing materials, such as hematite, and determine all the essential paramaters that enable us to model their performance. Realiable micro-kinetic models can subsequently underpin the finite element models that enable us to design up-scaled photoelectrochemical reactors. We are investigating the following aspects of reactor design:
- Optimisation of the mechanism for the illumination of one or more photoelectrodes (e.g. device tilt angle and/or use of optical components);
- Minimisation of ohmic losses between the photoelectrodes, as well as current density distributions across the photoelectrode surfaces (see image below);
- Efficient separation of the gaseous products of the water splitting process, minimising crossover;
- Effective capture of hydrogen, enabling accurate quantification of the solar to hydrogen conversion efficiency;
- Continuous operation under varying light intensities (what to do at night?).
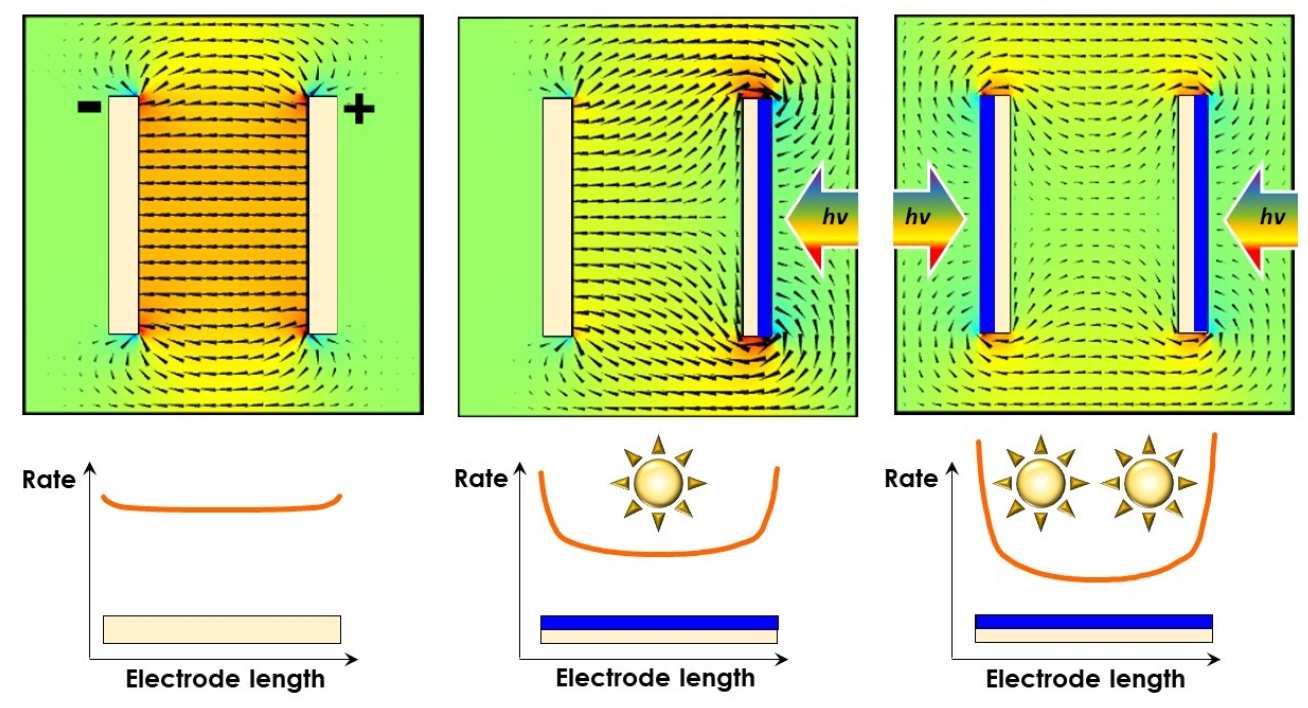
Problem in scale-up
Why do we use finite element models to help us design our devices? The illustration on the right shows, first, the ideal distribution of the electric field between a cathode and an anode of an electrochemical ractor - it is mostly uniform. This ensures a uniform ionic current between the electrodes, and hence a uniform reaction rate across the electrode surfaces. It is the ideal case scenario.
If the electroactive surface of one electrode is oriented away from the other electrode, the electric field becomes distorted (middle image). This effect becomes further exacerbated if both electroactive surfaces need to face away from each other (image on right) - this may be the case if both electrodes are photoactive and require irradiation by the full solar spectrum in order to function well.
These phenomena are extremely important in electrode/reactor scale-up and are examined closely in our group.
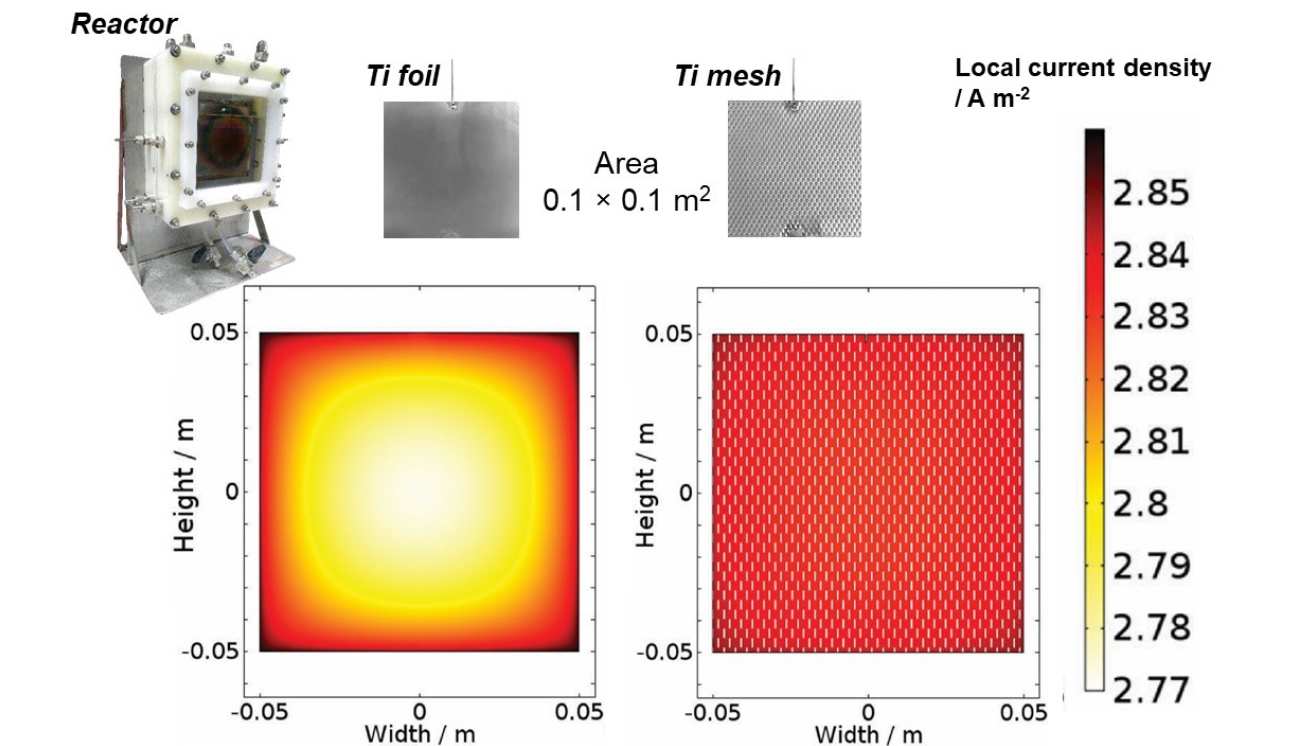
Reaction and reactor modelling
The image on the right is an extract from a study that was a first-of-its-kind, successful attempt to investigate experimentally and to model the micro- and macro-kinetics of photoelectrochemical water splitting in up-scaled reactors. Based on rigorous characterisation, we developed a semi-empirical model of water splitting kinetics at a hematite | NaOH interface; this model was incorporated into a finite element model of one of our up-scaled reactors and used to simulate reactor performance as a function of experimental variables. Different photoelectrode geometries were investigated to illustrate the impact of macro-design choices on the overall current density, which is a direct measure of the H2 (and O2) production rate. The resultant, experimentally verified, model accounted for the overall energy balance of a photo-electrochemical system as well as material properties, electrochemical and photoelectrochemical thermodynamics and kinetics at solid | liquid interfaces, ionic transport and the primary and secondary (i.e. allowing for electrode kinetics) current distributions between geometrically complex electrodes.
For more images from our photoelectrochemical reactor development projects please visit the Gallery.
Publications
-
Color-tunable hybrid heterojunctions as semi-transparent photovoltaic windows for photoelectrochemical water splitting - F. Eisner, B. Tam, V. Belova, W. Ow, J. Yan, M. Azzouzi, A. Kafizas, M. Campoy-Quiles, A. Hankin and J. Nelson, Cell Reports Physical Science, 2021, 2, 100676.
-
En route to a unified model for photoelectrochemical reactor optimization. II–Geometric optimization of perforated photoelectrodes - F. E. Bedoya-Lora, A. Hankin and G.H. Kelsall, Front. Chem. Eng., 2021, 3, 749058.
-
A review of inorganic photoelectrode developments and reactor scale-up challenges for solar hydrogen production - B. Moss, O. Babacan, A. Kafizas and A. Hankin, Adv. Energy Mater., 2021, 11, 2003286.
-
From millimetres to metres: the critical role of current density distributions in photo-electrochemical reactor design - A. Hankin, F. E. Bedoya-Lora, C. K. Ong, J. C. Alexander, F. Petter and G. H. Kelsall, Energy Environ. Sci., 2017, 10, 346.
-
Hydrogen sulfide splitting using solar energy and hematite photo-anodes - F. E. Bedoya-Lora, A. Hankin and G. H. Kelsall, Electrochim. Acta, 2019, 317, 384.
-
En route to a unified model for photo-electrochemical reactor optimization. I - Photocurrent and H₂ yield predictions - F. E. Bedoya-Lora, A. Hankin and G. H. Kelsall, J. Mater. Chem. A, 2017, 5, 2268
Webinar: Hydrogen Production Using Solar Energy
First aired 20 January 2015 via the Knowledge Transfer Network.
This technical presentation compares hydrogen production by photovoltaic powered electrolyser systems and photoelectrochemical water splitting systems.
Please note: the recording begins at 0:28 and there are two interruptions to the recording at 01:53 - 02:52 and 39:21 - 45:09 minutes.