Multifunctional performance metrics
Presentation or dissemination of multifunctional performance results using existing metrics to audience members with less expertise in multifunctionality often presents a challenge because the properties of multifunctional materials taken in isolation appear to have low performance compared to those of monofunctional counterparts. For instance, using traditional metrics, a structural battery with a specific energy of 110 Wh/kg may be considered to have a performance significantly below that of a conventional lithium ion battery (~200 Wh/kg). Similarly, the specific power or specific elastic modulus of structural supercapacitors may both appear too low to merit consideration ahead of conventional materials and devices. This perception of low performance stems from not taking a holistic viewpoint. Multifunctional efficiency indices [1-3] have been developed to capture the multifunctionality to some degree and presenting system-wide mass or volume savings can also give a better indication of multifunctional performance than looking at specific energy or elastic modulus alone. However, these approaches do not present the results in the way end-users would prefer to see them such that end-users can interpret and compare the results with respect to familiar material properties.
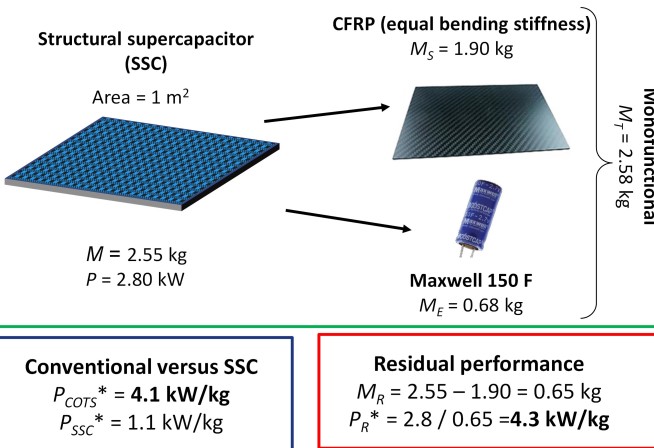
Instead, we have developed a new way to present multifunctional performance results, using ‘residual specific’ properties [4]. To demonstrate this methodology, residual specific properties have been determined for two cases; a structural battery and a structural supercapacitor. Performance data from previously published models [5] was used to determine a structural battery with the same global bending stiffness of a conventional composite panel to have a residual specific energy of 409 Wh/kg. Similarly, this method was applied to structural supercapacitors and yielded a residual specific power of 4.3 kW/kg which slightly exceeded that (4.1 kW/kg) of a comparable size commercial off-the-shelf supercapacitor (Figure 1).
Multifunctional design study into aircraft cabin applications
A theoretical investigation has been carried out to consider the potential use of structural power composites in regional aircraft passenger cabins and the corresponding challenges to widespread use, including fire resistance, long-term cycling performance and cost [6]. This study focused on adapting sandwich floor panels with structural power composite face sheets, designed to power the in-flight entertainment system. This investigation selected a widely-used aircraft (A220-100) as a case study platform to demonstrate the methodology and determined the mechanical, specific energy and specific power requirements for this application, as well as the weight, volume and emissions savings achievable. The proposed multifunctional design methodology consisted of five main stages: (1) Define performance requirements to determine the feasibility of using multifunctional materials; (2) Explore how best to integrate multifunctional materials into new systems, configurations or architectures; (3) Select a multifunctional system configuration using a simplified analysis based on the requirements of the proposed application; (4) Understand how integrating a multifunctional material affects the design of the associated mechanical and electrical systems; (5) Evaluate the benefits and challenges of using multifunctional materials for a given application. Structural modelling of state-of-the-art laminated structural power composites was used to define the mechanical requirements. The mass of composites needed to meet these mechanical requirements and audits of the total energy and power demands were used to calculate electrochemical energy storage performance targets.
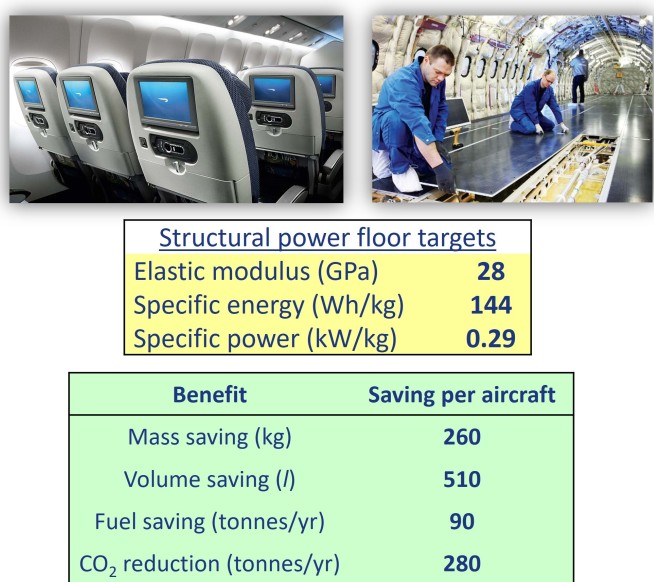
For an Airbus A220-100, the analysis predicted potential mass and volume savings of approximately 260 kg and 510 l and annual reductions in CO2 and NOx emissions per aircraft of approximately 280 tonnes and 1.2 tonnes respectively, provided the structural power composite could achieve the following properties: specific energy > 144 Wh/kg, specific power > 0.29 kW/kg, in-plane elastic modulus > 28 GPa and in-plane tensile and compressive strengths > 219 MPa (Figure 2). These performance targets can help researchers to develop and tailor structural power composites for this application by guiding the selection and optimisation of the material constituents and fabrication processes. Significantly, in this cabin application, the use of a distributed energy storage system offers a significant range of mass and cost savings associated with a simplified electrical distribution system design and the use of ground-generated electrical energy rather than drawing power from the engines via a generator. This extended design analysis of a specific component highlighted both the far-reaching implications of implementing structural power materials and the potential extensive systemic benefits.
Multifunctional design study into commercial electric aircraft applications
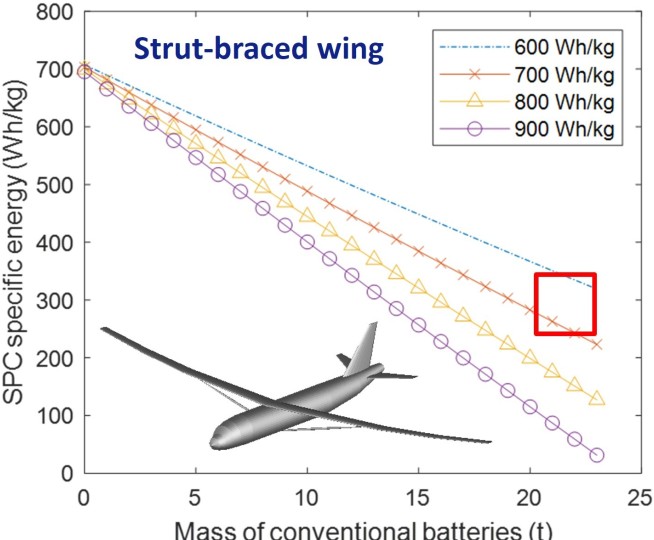
The future of commercial aviation is driven by the need to improve efficiency and lower emissions. All-electric aircraft present a route to eliminating direct fuel burn emissions, but their development is stifled by the limitations of current battery energy and power densities. Hence, another multifunctional design study investigated the feasibility of integrating structural power composites into future electric aircraft and assessed the potential impact on greenhouse gas emissions up to 2050 [7]. Using the Airbus A320 as a platform, three different electric aircraft configurations were conceptually designed to incorporate three synergistic technologies: structural power composites, slender wings and distributed propulsion.
Specific energy and power targets for the structural power composites were estimated by determining the aircraft mission performance requirements and structural weight. Compared to a conventional A320 over a 1500 km journey, a parallel hybrid-electric A320 with structural power composites (> 200 Wh/kg) could potentially increase fuel efficiency by 15%. Structural power composites (> 300 Wh/kg) to supplement a battery-powered A320 could nearly halve the specific energy or mass of the batteries needed to power a 1000 km flight (Figure 3). Thus, structural power composites could facilitate the all-electric aircraft by lowering the high battery energy density targets and potentially enable lighter and safer electric aircraft.
Multifunctional design study into air taxi applications
The emergence of urban air travel is leading the aviation sector towards a more flexible and sustainable future, driven by electric vertical take-off and landing (e-VTOL) aircraft with new technologies and electric propulsion systems. A multifunctional design study investigated the viability of using structural power composites to supplement or replace batteries within electric air taxis, specifically, the CityAirbus, a four-seater eVTOL [8]. A typical mission profile was modelled and used to determine the total power and energy requirements for every flight segment. The mass of structural composites eligible for substitution with structural power composites was determined by a combination of structural analysis and a mass audit. The target values for the mechanical requirements of the structural power composite were determined based on the critical loading case likely to be experienced by the CityAirbus. Reduced structural power composite mechanical properties relative to conventional structural composites were considered for bending and in-plane loading to determine target values associated with increased thicknesses needed to carry the loads.
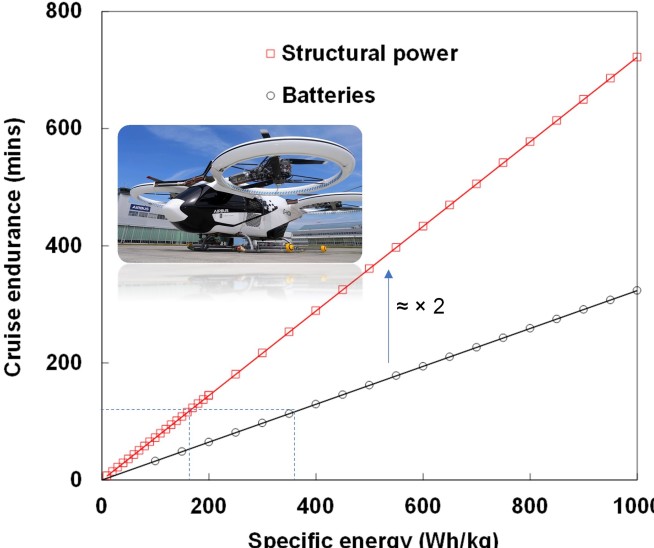
Various scenarios with different take off masses and structural power composite masses were simulated to determine the structural power composite target performance values to meet the requirements of the CityAirbus mission profile. These targets were compared to those for batteries (Figure 4). If all of the eligible composite mass and batteries were to be replaced by structural power composites, the CityAirbus weight could be reduced by 25%. Furthermore, the cruise endurance could be approximately doubled by replacing batteries with structural power composites having the same specific energy. This study into structural power composite applications in the CityAirbus indicated that the urban air transport sector presents an promising adoption route for structural power composite technology. Furthermore, structural power composites have the potential to aid the commercialisation of air taxis by making the range more attractive to potential customers or allowing greater payloads to be carried by reducing or removing batteries.
References
- Snyder JF, et al. Performance metrics for structural composites with electrochemical multifunctionality, J. Compos. Mater. 2015, 49.
- O’Brien DJ, et al. Design and performance of multifunctional structural composite capacitors J. Compos. Mater. 2011, 45, pp. 2797–809.
- Freund R, et al. Multifunctional efficiency: extending the concept of atom economy to functional nanomaterials, ACS Nano, 2018, 12, pp. 2094–105.
- Johannisson W., et al. ”A residual performance methodology to evaluate multifunctional systems”, Multifunctional Materials, Vol 3(2), 2020, 025002 (open access).
- Johannisson W, et al. Model of a structural battery and its potential for system level mass savings, Multifunct. Mater. 2019, 2, 035002.
- Nguyen SN, et al. Conceptual multifunctional design, feasibility and requirements for structural power in aircraft cabins, AIAA J Aircraft, 2021, Vol. 58, No. 3, pp. 677-687.
- Karadotcheva E, et al. Structural power performance targets for future electric aircraft, Energies 2021, 14(19), 6006 (open access).
- Ishfaq A, et al. Multifunctional design, feasibility and requirements for structural power composites in future electric air taxis, J. Compos. Mater. 2022, 57, 4.
Related links
Contact
Professor Emile S Greenhalgh
Department of Aeronautics
Imperial College London
South Kensington Campus
London SW7 2AZ
+44 (0)7958 210 089
e.greenhalgh@imperial.ac.uk