Carbon capture and storage at Imperial
by David Silverman and Mia Roberts
On Saturday 11 May 2019, the Mauna Loa Observatory in Hawaii – the oldest continuous CO2 monitoring station – took a historic measurement. On that day, which also saw temperatures reach 29 degrees Celsius near the entrance to the Arctic ocean, the observatory measured 415 parts per million of CO2 in the Earth’s atmosphere – more than there has been for 800,000 years.
The evidence that increasing levels of CO2 in the atmosphere are changing the Earth’s climate is overwhelming, and there is a recognised need to limit global warming in order to stave off the worst impacts on health, ecosystems, economies and resources. In 2015, 195 nations made an unprecedented commitment through the Paris Agreement to limit the global temperature rise to 1.5–2⁰C above pre-industrial levels. More recently, the UK made a legal commitment to reach net zero CO2 emissions by 2050 – just three decades from now. So how can society best fulfil these important commitments in the limited time available?
The challenge of eliminating fossil fuels
Geoffrey Maitland CBE, Professor of Energy Engineering in Imperial’s Department of Chemical Engineering, says that renewable energy and other zero carbon energy sources are crucial for combating climate change, but cannot be rolled out quickly enough to meet increasing energy needs around the world. “Some countries will implement renewables quickly,” he says. “However, much of the developing world already suffers from energy poverty, and the population is growing. Renewables will not be implemented quickly enough worldwide to meet the growing demand.”
Even if all energy came from renewables, he adds, fossil fuels would still be required to meet demand for other industrial processes: “We use fossil fuels not only for energy but also for cement and steel production, which are major carbon emitters. It’s very difficult to change those processes.”
This means, Professor Maitland says, that non-fossil energy sources cannot by themselves limit climate change to the extent needed: “If we convert to renewables as quickly as people reasonably predict we can, and we become as energy efficient as possible, and we build nuclear plants at the maximum possible rate and overcome the political problems with that, we’ll still be short of meeting the Paris target.”
Professor Nilay Shah, head of the Department of Chemical Engineering, makes a related point. “Even with the massive reduction in CO2 emissions that renewables will enable, we'll still be emitting CO2, so they’ll never be enough by themselves to stabilise the climate,” he says. “All that will happen is the CO2 concentration in the atmosphere will increase more slowly. We need to get to net zero emissions and eventually perhaps net negative for some time.”
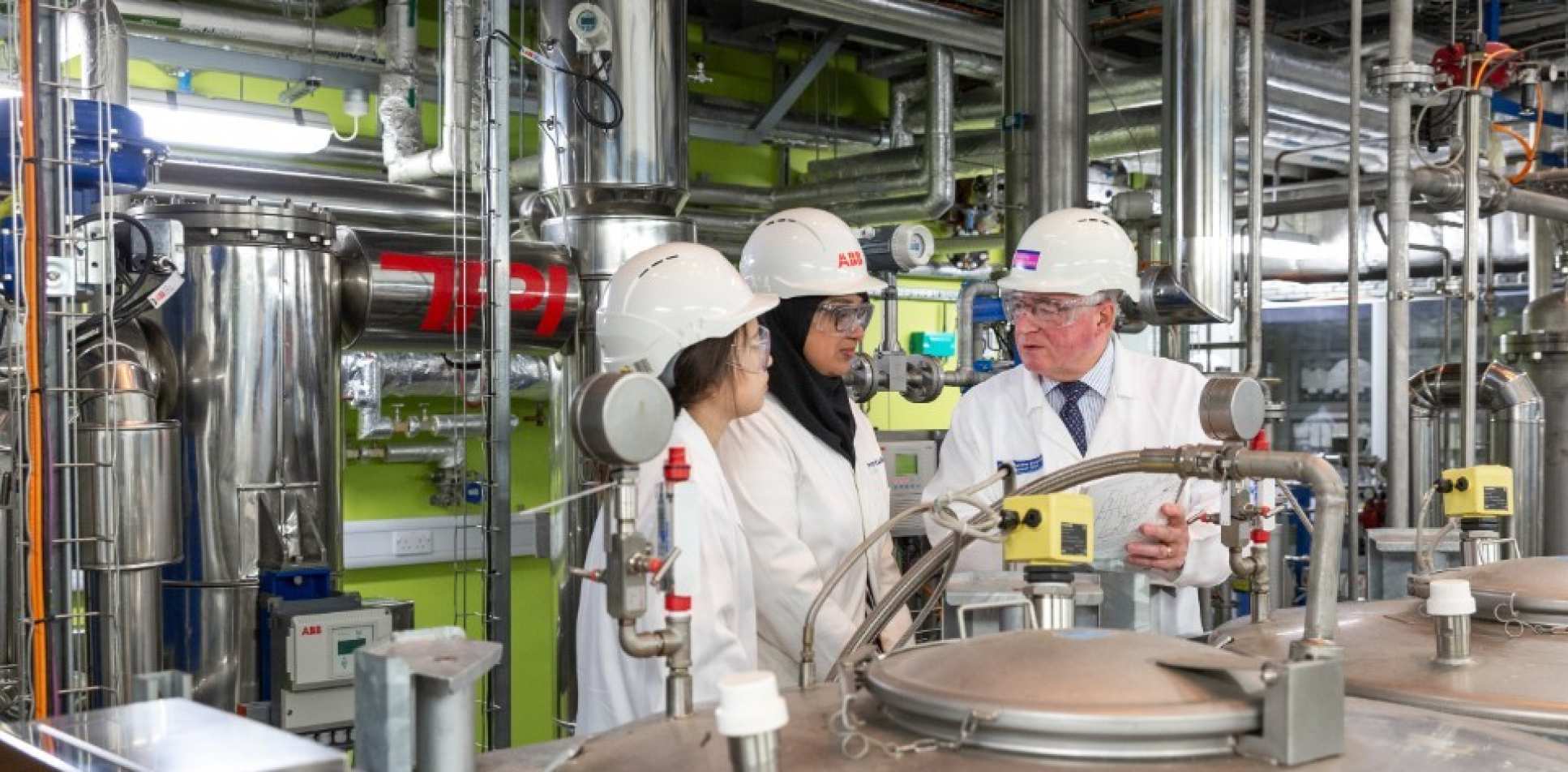
Carbon capture and storage
Carbon capture and storage (CCS) is a set of technologies intended to help tackle climate change by capturing CO2 and storing it so it cannot enter the atmosphere. This typically involves capturing CO2 from power plants and factories and injecting it into the pores of rocks deep underground, reducing the net emissions of fossil-based energy production and industrial processes.
Combined with bioenergy, CCS could contribute to net negative emissions. In a bioenergy with CCS (BECCS) chain, CO2 is absorbed from the atmosphere into plant materials via photosynthesis. The biomass is then used for energy in power plants, industrial facilities and biorefineries, which deploy carbon capture technologies to make the process as a whole carbon negative. The most popular methods of carbon capture are pre combustion, which involves promoting a series of reactions to produce hydrogen and a pure stream of CO2 from a hydrocarbon fuel prior to combustion; post combustion, which uses solvents to separate CO2 from a flue gas after combustion; and oxy combustion, which involves burning fuel in pure oxygen, instead of air, to produce a flue gas composed mostly of CO2 and water vapour.
These processes are not new. Dr Niall Mac Dowell, Reader in Energy Systems in the Centre for Environmental Policy, notes that oxy combustion was developed by Carl von Linde in 1895 and brought to market in 1905, while the post combustion process was first patented in the early 1930s.
The techniques involved in CO2 storage date back to the 1970s, when oil and gas companies pioneered a method of injecting substances such as CO2 into the pores of rock formations kilometres underground to recover the hard-to-extract oil that was stored in them. Fortuitously, at the pressure and temperature ranges involved, CO2 enters a supercritical state in which it has the viscosity of gas, making it easy to inject, but the density of liquid, making it efficient to store. Since 1996, the technique has been used to inject CO2 into places like saline aquifers for the sole purpose of keeping it out of the atmosphere. There are currently 12 geological CO2 storage sites of this kind worldwide.
Following the continuing work that has been done on CCS in recent decades, Anna Korre, Professor of Environmental Engineering in the Department of Earth Science and Engineering and Co-Director of the Energy Futures Lab, says that CCS offers an established method for keeping CO2 out of the atmosphere over very long timescales. “The key technologies used in CCS are already proven,” she says. “The focus of research at Imperial College London is therefore on maximising the efficiency and effectiveness of CCS, fine tuning our understanding of risk, and developing economics and policy proposals that will help organisations and policymakers understand the appropriate role that CCS technologies should play alongside other technologies in the coming energy transformation.
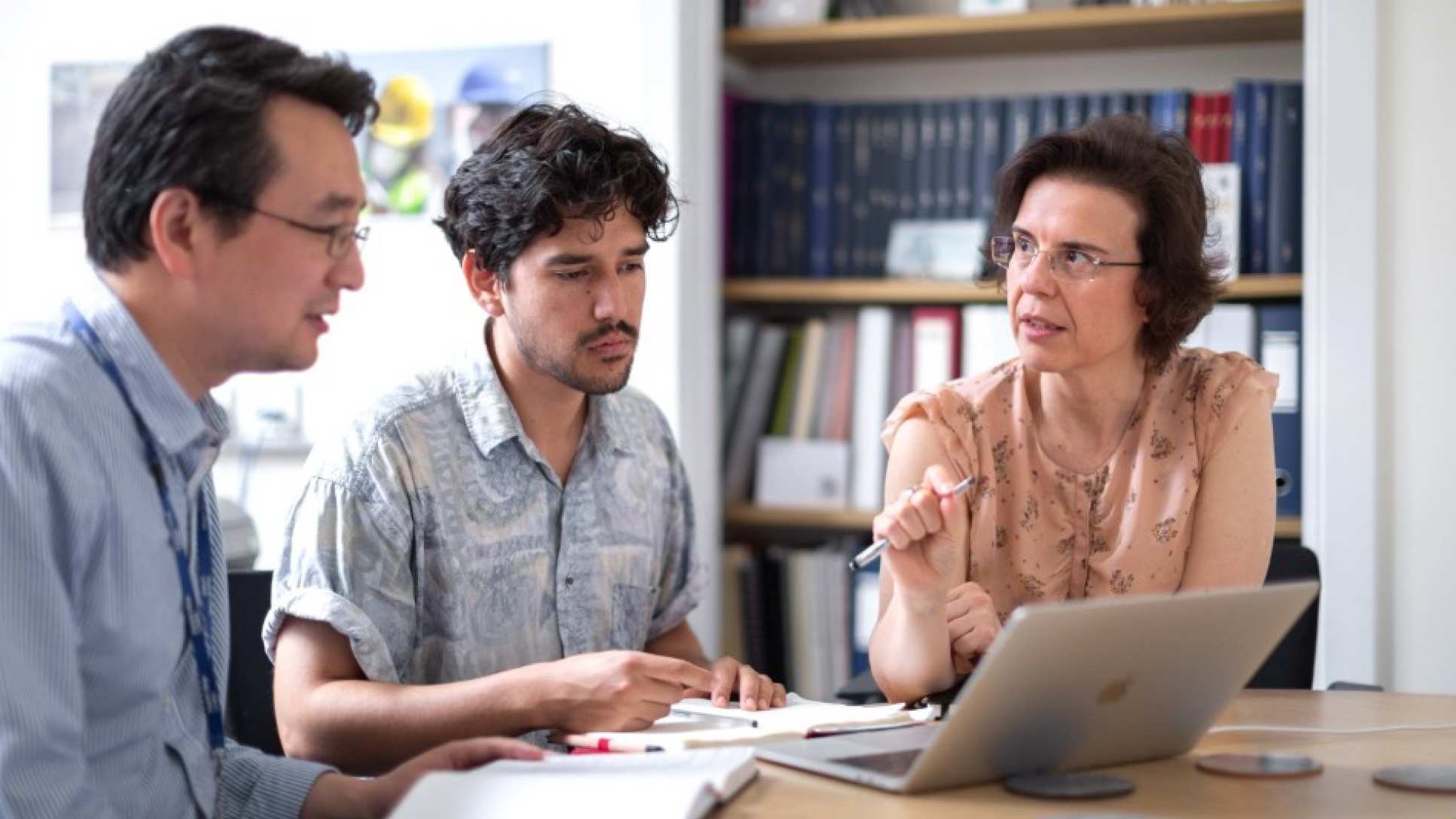
Improving efficiency in capture
One of the main challenges associated with carbon capture is efficiency, as the process itself has a parasitic energy demand on the system it is applied to. Professor Paul Fennell of Imperial’s Department of Chemical Engineering is exploring this challenge with a focus on industry. Industrial processes such as cement, iron ore smelting and steel manufacturing account for approximately 45 % of direct emissions from large plants worldwide and, as yet, there are no alternative ways to produce these heavily relied-upon materials. Professor Fennell and his team are exploring new technologies for carbon capture and designing tailored, scalable and efficient CCS systems for these major emitters.
“The main disadvantage of standard carbon capture technology – monoethanolamine scrubbing – is that it takes a lot of energy away from your power station or manufacturing plant to run the capture process. So we are looking at technologies that would more than halve that energy penalty or, in some cases, eliminate it altogether. I began by looking at how to integrate the most efficient CCS system into a cement plant, using a technology called calcium looping, which we successfully demonstrated in collaboration with colleagues in the EU CaOling project.” In calcium looping, calcium oxide (CaO) from limestone (CaCO3) reversibly reacts with CO2 in the flue gas to produce a pure stream of CO2. “Because it’s a reversible reaction, we get our calcium oxide back, which we can reuse again and again.”
Professor Fennell is also working on the demonstration of Direct Separation technology, which enables pure CO2 to be captured as it is released from the limestone itself – where two thirds of the emissions are generated – without significant energy or capital penalty.
“My group does a lot of work validating the kinetics of reactions going on in CO2 capture systems,” he says. “We have globally leading experimental equipment for doing high pressure, high temperature work. We study the rate of a reaction at micro scale, for example to see how fast a gram of sorbent will take up a particular amount of CO2. Then we scale that up so we can think about designing large chemical reactors. Our work ranges from the experimental – thinking about new processes – to testing the materials, all the way up to pilot demonstration.
“We also look at how things fit into the global decarbonisation agenda. For example, working with Imperial’s Grantham Institute – Climate Change and the Environment, we might look at how far we can push to decarbonise cement, and how that might affect how deeply we need to decarbonise air travel. It’s a holistic perspective that industry alone may not be able to take, but, as an academic institution, we can provide.”
Carbon dioxide storage
Once CO2 has been captured, it needs to be permanently prevented from entering the atmosphere. A lot of work at Imperial is focused on better understanding how CO2 flows through rock formations after it has been injected, allowing researchers to make better informed judgements about the maximum speed and quantity at which CO2 can be safely injected at a given site. This makes geological storage more efficient, and reduces the risk envelope, making projects more commercially viable.
Imperial researchers are at the forefront of a new approach to this that uses X-ray tomography to experiment on samples from rock formations. “We take rocks and put them in a type of reactor called a core holder, which allows us to inject fluids into one end of the cylindrical rock sample and retrieve fluids from the other side”, explains Dr Sam Krevor in the Department of Earth Science and Engineering. “We do this in a setup that allows us to recreate the pressures and temperatures we see underground, inside an X-ray scanner so we can image the movement and trapping of CO2 in 3D.”
This work has resulted in some surprising discoveries. “It was assumed that water and oil have separate flow paths,” says Professor Martin Blunt, Chair in Petroleum Engineering in the Department of Earth Science and Engineering. “What we found was something much more dynamic. Periodically CO2 would invade a region normally filled with water to get along. Like when pedestrians take the road over to get from one side to the other,” says Professor Blunt. “This was not what we previously thought.
Having a secure understanding of fluid flow is important when it comes to CO2 injection for preventing climate change, because it helps you to guarantee that it’s going to stay underground.”
Researchers like Professor Blunt, Dr Krevor and Professor Maitland aim to understand fluid flows through rocks like sandstone at the pore scale. They and others including Professor Korre and Professor Sevket Durucan in the Department of Earth Science and Engineering are also applying the technique to understand CO2 flows at reservoir and regional scales. “We at Imperial have become the leading centre in the world for understanding carbon storage at the mechanistic pore-scale level and linking that to upscaled models that include reservoir and regional models,” says Professor Maitland.
The challenge at these higher scales is accounting for the varying properties of the rocks at different depths and in different locations with access to a finite number of samples. The researchers do this using geostatistics and Kalman filtering, approaches that improve upon the accuracy of commercial reservoir modelling by accounting for the spatial characteristics of the data. “These models benefit quite a lot from the geologist’s expert understanding of the subsurface, as well as the mathematical skills needed to convert this into an input that can go into a reservoir simulator that evaluates different scenarios and possibilities, and identifies which are the most likely,” says Professor Korre.
“We try to account for the rock’s heterogeneity, which is often the result of fractures. We look at the strength and mechanical properties of the rock around the well. And we use all that information to understand the integrity of the reservoir, the injection pressures it can safely take, and the rate at which we can inject,” says Professor Durucan.
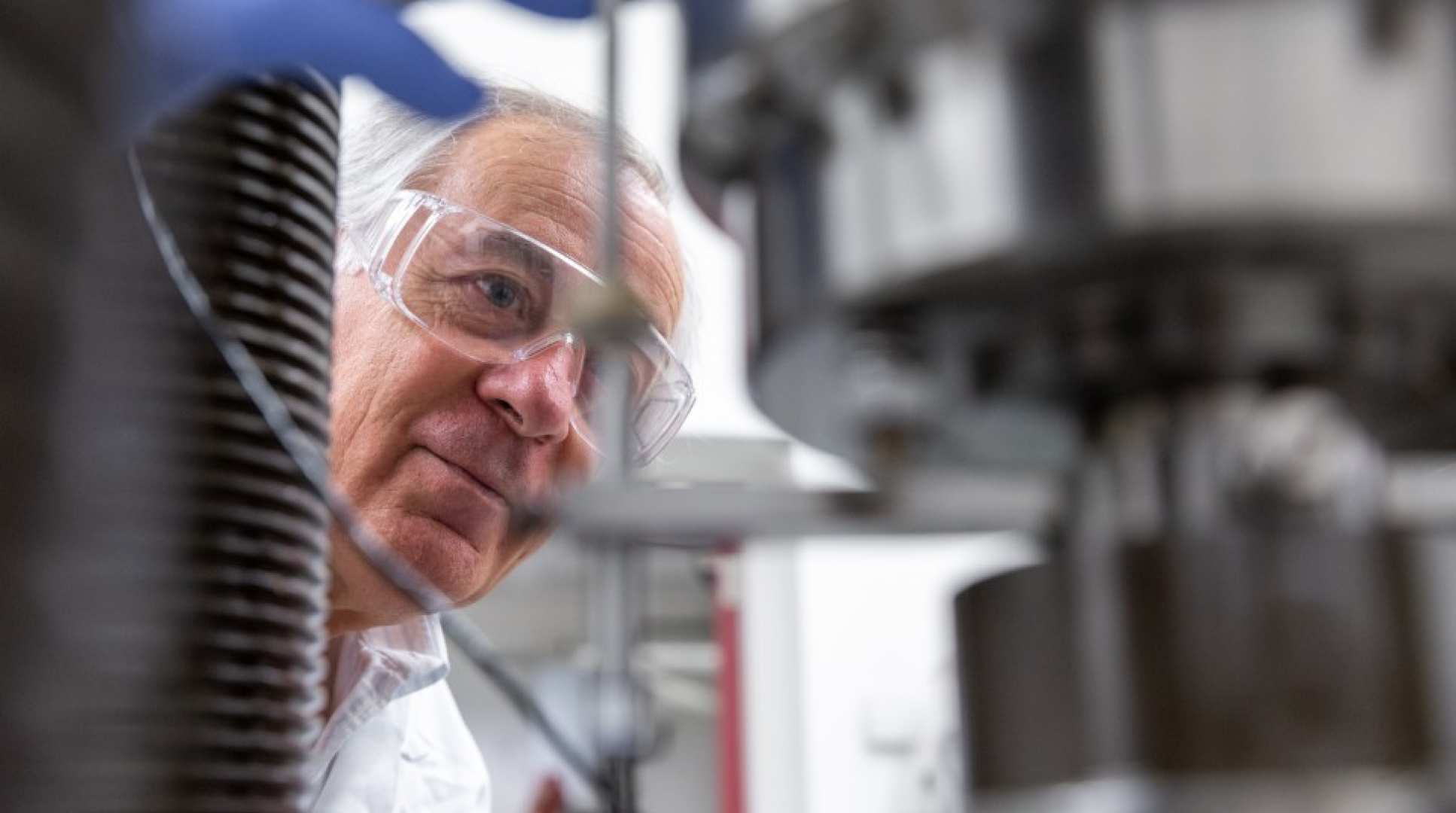
The right energy mix
Dr Mac Dowell examines the kinds of power systems, industrial systems and infrastructure we need in order to transition to a low carbon global economy in a financially-viable but also equitable way.
This work helps policymakers understand how they can meet environmental goals such as the Paris Agreement alongside economic goals such as the Sustainable Development Goals agreed at the UN (e.g. zero hunger). Dr Mac Dowell says: “Usually we think about how to transition to low carbon at least cost. But another way to think about it is max value. For example, nuclear power is an effective way to reduce CO2 emissions, but in Britain we mostly buy nuclear power plants from other countries. Once we think about things through the max value lens, the UK bioeconomy – for example BECCS – starts to become more important, because it creates more jobs and prosperity here”.
Through this lens, Dr Mac Dowell is developing a more tangible account of how an energy transition could take place. “Up until now, the tools didn’t exist to account for technology transitions while also thinking about macroeconomics and how thatL transition might work. Until now people just haven’t thought about it that way.”
One tool that Dr Mac Dowell’s group is developing is an optimisation algorithm called MONET (Modelling of Negative Emissions Technology). The algorithm allows a user to input a set of goals, which might include maximising energy production, minimising cost or minimising net CO2. It then performs calculations based on factors such as the costs and environmental footprints of CCS technologies like BECCS, afforestation and enhanced weathering, and the geographical distribution of available water, land, biomass and CO2 storage capacity, while accounting for constraints like the need for drinking water and agricultural land. It also accounts for the complex synergies that come into play as the infrastructure evolves, for example the positive effect that biomass production has on water availability. The algorithm finally outputs a model of a global energy system optimised to meet the chosen goals.
Modelling work is also helping researchers to understand the costs and benefits of CCS projects that are currently under consideration. Professor Korre carries out life-cycle analyses that track their environmental effects, including their net emissions of CO2, other greenhouse gases like methane, and other environmental effects, for example on water supplies. “For us, the start is the raw materials,” she explains. “We follow the raw materials through their production cycle, then their utilisation cycle, accounting for emissions, CO2 and waste generated, then the process of transporting, injecting and storing CO2, to close the whole loop.”
By accounting not only for the emissions released locally by power stations but also, for example, for the methane released during natural gas production and coal mining and the CO2 emitted when transporting raw materials, Professor Korre and her group can calculate the net emissions of a CCS system in a very accurate way that is used by industry in emissions performance reporting and reconciliation. In a similar fashion, Professor Korre analyses the economic costs of each stage in the life-cycle.
An important obstacle to mass implementation of CCS is that the necessary economic incentives are not currently in place. Drawing on both the life-cycle analysis and life-cycle costing, her team carries out a real options analysis. “We actually consider the economic incentives that would be required to make CCS a reality”, Professor Korre explains. “For example, whether the government needs to provide a subsidy on the CO2 price, invest upfront when the site is being evaluated to reduce the uncertainty and minimise the financial risk of the project. Then we study the techno-economics of the system, considering the geology, the engineering system performance, and the overall environmental and financial performance of the system.”
Piecing together the full picture
“The problem of climate change will never be solved with incremental approaches,” says Professor Shah, who is a leading expert in the design and analysis of energy systems. “Sooner or later we have to have a complete transformation of our energy and industrial system.” This kind of transformation hinges on collaboration – academia, industry, governments, businesses and communities from all around the world working together to bring about change at local, national and global levels. “The word consolidation comes to mind, when I think about the direction we need to move in,” adds Dr Mac Dowell. “As a society, we’re very good at looking at elements of the sustainable transition in isolation, but we’re very poor at looking at them in combination and from the perspective of the integrated whole.”
It’s a perspective that Imperial is helping to bring by connecting people from across disciplines, sectors and continents and providing objective information on all elements of CCS from the micro to macro scale. From discussing the latest policy-relevant findings with civil servants, to working with EU partners on large-scale demonstration projects, to establishing international research programmes looking at the storage potential of Middle Eastern reservoirs, Imperial is at the heart of an international network working to implement the most promising technology and systems for safely and effectively decarbonising the economy.
Working in partnership
ELEGANCY: enabling a low-carbon economy via hydrogen and CCS
Despite the potential value of CCS, there has been a disconnect between policy ambitions, technology readiness and industrial uptake. This, some experts say, is mainly due to the lack of a business model: costs are immediate, but the benefits are longterm. Similarly, the introduction of hydrogen as a low-carbon fuel has been slower than expected. Imperial is the UK lead partner for ELEGANCY, a research project involving consortia from the UK, Norway, the Netherlands, Germany and Switzerland. ELEGANCY’s aim is to make CCS more economical by linking it with the production of hydrogen for energy, thereby overcoming one of the main obstacles to investment by industry and government while supporting the rapid introduction of introduction of hydrogen as an energy carrier. The research is carried out by a multidisciplinary team led by Imperial’s Professor Martin Trusler, Professor Nilay Shah, Dr Ronny Pini, Dr Sam Krevor and Dr Niall Mac Dowell.
LEILAC: Low emissions intensity lime and cement
Cement and lime manufacture are responsible for nearly 10% of man-made CO2 emissions. These two processes are unusual because most of their emissions come not from fuel combustion but the calcination of limestone (CaCO3), producing lime (CaO) and CO2. LEILAC is a €21 million project developing a new method of calcining limestone based on Direct Separation technology. Represented by Professors Nilay Shah and Paul Fennell, Imperial is part of a unique, international consortium including research groups, energy and minerals processing companies, modelling software providers, life-cycle assessment consultancies and one of the world’s largest building materials manufacturers, Heidelberg Cement. Together, the group successfully demonstrated Direct Separation technology at a large-scale pilot plant in Lixhe, Belgium, showing its capability to support Europe in achieving CO2 emission targets in a timely, effective and efficient manner.
The Qatar Carbonates and Carbon Storage Research Centre
Approximately half the world's reservoirs are carbonate, including large Middle Eastern reservoirs in Saudi Arabia, Kuwait and Qatar, which hold the potential to store significant quantities of the world’s captured CO2. However, carbonate reservoirs are much less understood than sandstone reservoirs, due to their complex structure and the fact that carbonates can be dissolved by CO2 in water. The Qatar Carbonates and Carbon Storage Research Centre (QCCSRC) was a ten-year, $70 million strategic collaboration between Imperial, Qatar Petroleum, Shell and the Qatar Science and Technology Park (part of Qatar Foundation) that revolutionised knowledge of these reservoirs. Founded by Professor Geoffrey Maitland, the QCCSRC saw the creation of five specialised carbon storage laboratories, which welcomed approximately 50 PhD students and 30 postdoctoral researchers in its ten years, making it a leading centre for training the next generation of CCS experts.
LCA-OGCI and ALIGN-CCUS projects
Any effective, sustainable energy portfolio of the future will likely include a range of technologies and energy sources. Being able to objectively compare different options is crucial to making informed decisions about the best route forward. Professor Anna Korre and Professor Sevket Durucan are leading the first research project awarded by the Oil and Gas Climate Initiative conducting a techno-economic assessment of greenhouse gas mitigation options in natural gas production, processing and liquefied natural gas transport value chains. Different facilities around the world are evaluated using Imperial’s life-cycle assessment modelling tool (ICLCA) and their performance, both in their current state and with the implementation of CO2 storage at the end of the process are compared. In the ALIGN-CCUS project, 34 science and industry partners including Imperial are working to transform six European industrial regions into economically robust, lowcarbon centres by 2025. A key project aim is to draw up blueprints to deliver carbon capture, usage and storage in the industrial regions of Teesside and Grangemouth in the UK; Rotterdam in the Netherlands; North Rhine-Westphalia in Germany; Grenland in Norway; and Oltenia in Romania.