Project 4
- Demonstrate attosecond transient absorption spectroscopy in the condensed phase.
- Introduce and exploit new high transmission and low-dispersion micro and nanoscale targets for attosecond science.
- Develop high harmonic spectroscopy on surfaces.
- Test and exploit ‘infrared-field-streaked’ nonlinear spectroscopy in bulk.
Current Research
- Development of a beamline for attosecond spectroscopy experiments
- Laser dressed transient absorption
- Versatile magnetic bottle electron spectrometer compatible with gas, liquid and solid phase matter experiments
- Sub-cycle transition dynamics in periodic structures
Introduction
An important topic in attosecond science is the investigation of valence and inner valence electron dynamics in molecules and condensed phase systems. Conventional HHG spectroscopy is a technique which provides excellent time resolution on the relevant timescales but the tunnelling nature of the ionization limits it to the investigation of outer-lying orbitals and the strong field can distort the dynamics being studied. The two techniques below offer solutions to this limitation.
Experiment
1. ATA (attosecond transient absorption) is a technique where a pump pulse is used to prepare a target system and a second delayed pulse is used to follow the time evolution of the prepared system by monitoring its delay dependent soft x-ray absorption spectrum. Contrary to earlier studies in atoms [1], our new beamline extends this study to real- time, state-resolved observation of ionization in aligned molecules. Moreover, the technique will be implemented to study electron dynamics in condensed phase targets (including clusters, solid foils/films, and liquids).
2. XiHHG (XUV initiated high harmonic generation). Instead of using the IR field to tunnel ionise the electron wave packets as the first step of HHG, we are investigating driving the ionisation with XUV attosecond pulses and recombining with IR pulses which may access deeper bound states [2]. This scheme allows the IR field intensity to be kept to a minimum while the probed hole states are selected by the energy of the XUV attosecond pulses.
Specifications
The laser system used for this beamline is KM Lab’s Red Dragon with 30 fs pulse duration, 8 mJ pulse energy at 1 kHz repetition rate centred at 790 nm. A HE TOPAS is utilised to reach the MID-IR wavelengths up to 2200 nm. The idler has a passively stable carrier envelope phase [3] which is compressed towards the few-cycle limit using hollow-core fibre compression technology.
This beam is sent into a vacuum chamber where it is split into three arms. This interferometer is on a bread-board mounted directly from optical table, decoupled from mechanical vibrations of pumps. The first arm produces high flux XUV attosecond pulses by phase-matching the HHG process with pressure tuning and wave-guiding in a hollow-core fibre [4]. Efficient XiHHG requires a flux on the order of 106 photons per pulse at 15-50 eV. The second arm is delayed relative to the first with a delay stage of nanometer resolution, which corresponds to a time resolution of ~7 as. This arm is actively stabilised to the first arm via a PID to monitor and correct for the drift in beam paths at 500 Hz. Also in this arm is a mechanical chopper to rapidly alternate between recording pumped and un-pumped signals to significantly improve signal-to- noise. The third arm is separately delayed to the first two by a delay stage and collinearly overlapped with the second arm by a beam combiner. The HHG arm and the pump arms are then recombined collinearly by a holey mirror.
Once the beams are recombined, they are imaged to a second gas jet, the target, by a 1 meter focal length toroidal mirror. Finally, the spectrum is dispersed by a flat field spectrometer with a detector comprising of 12.3 mm Andor X-ray CCD camera, or a 40 mm MCP with a phosphor screen that is imaged by a camera.
Current researchers
S.E.E. Hutchinson, A. Sanchez-Gonzalez, L. Miseikis, T. Siegel, Z. Diveki, E. Simpson, J.P. Marangos.
References and relevant links
[1] Wirth, A. et al. Science 334, 195–200, (2011).
[2] Gademann, G. et al. NJoP, 13, 033002, (2011).
[3] Balčiūnas, T. et al. OSA CLEO/QELS (2010).
[4] Popmintchev, T. et al. PNAS, 106, 10516–10521, (2009).
Introduction
The natural timescale of intra atomic/molecular electron dynamics is on the attosecond level. Producing light pulses with a comparable duration is required in order to study those ultrafast phenomena. High harmonic generation (HHG) is up to now the most developed attosecond light source. HHG is a laser field driven process that requires strong field ionisation as the initial step, a requirement that means a high IR laser intensity is needed.
In order to overcome the problem that the high intensity IR field may perturb the target atom and induce changes in the electronic structure during the HHG, XUV initiated HHG (XiHHG) was proposed [1]. In this scheme, instead of tunnel ionization, the electrons are ejected by single photon ionization by prepared XUV radiation and a delayed IR field forces these electrons to turn back and recombine with the core. Existing experiments show that new harmonics can be generated with this process which were not present in the original XUV beam [2]. Furthermore, using photoionization in the first step allows to study core orbitals without inducing valence electron dynamics.
Experiment
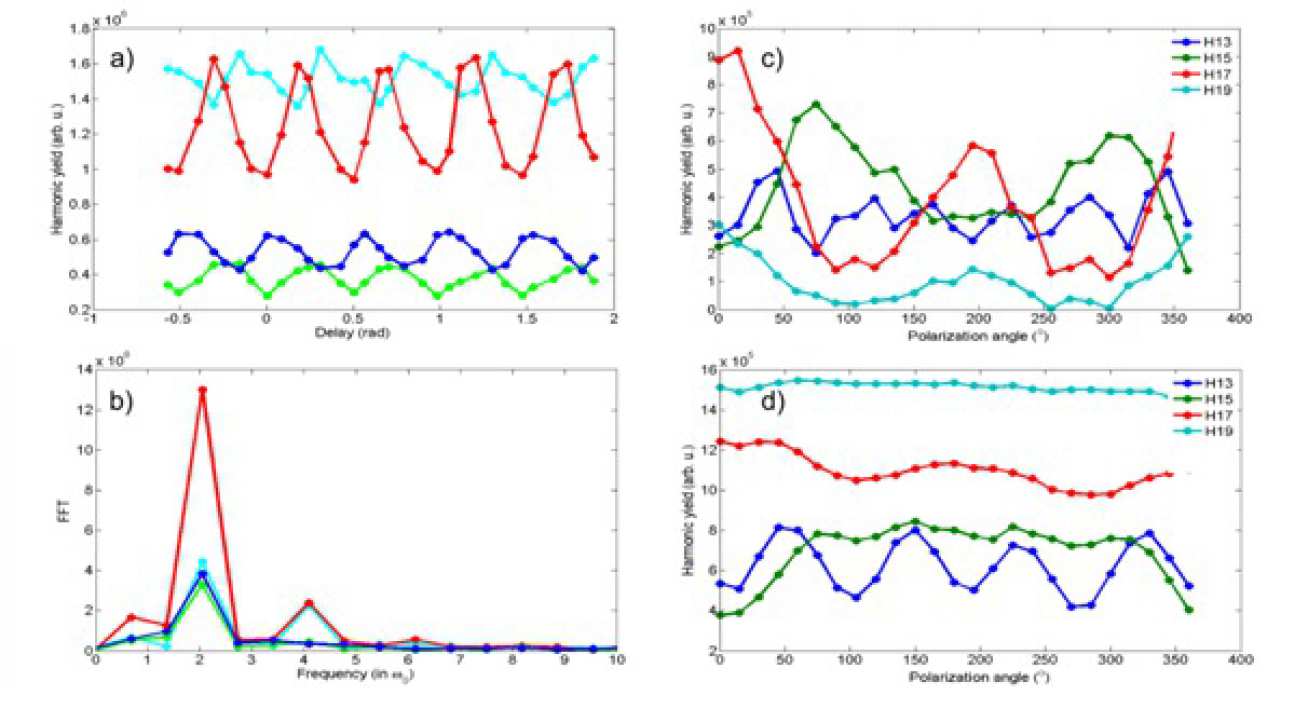
To investigate XiHHG experimentally and to conduct other transient absorption experiments, we have developed a new beamline (see Development of a beamline for attosecond spectroscopy experiments) that allows us to perform pump probe measurements with IR and XUV beams. We generate harmonics in Kr. Along with an IR beam they can be focused into a He target. Their combined presence results in a modulated XUV absorption that is observed by a flat field spectrometer at the end of the beamline.
To investigate the observed absorption oscillation (an example is shown in Fig 1a), we can control the intensity, polarization and ellipticity of the IR beam. Fig 1b shows the Fourier analysis of a typical IR induced absorption oscillation revealing strong 2ω and 4ω oscillations, where ω is the IR frequency. Depending on the intensity of the IR beam there are different picture that can be used to explain this, including XiHHG (intensity >> 1013 W/cm2 viewing the continuum-continuum transitions dri ven by the laser field in the strong field approximation) and IR laser induced absorption modulations (intensity < 1013 W/cm2 where the continuum-continuum transitions are best viewed in terms of lowest order multi-photon transitions, more details in [3,4]).
The results of polarization (and other parameter) scans (Fig 1b and d) hint that we are observing a form of IR induced absorption. This is supported by the absence of higher order harmonics that can be generated in an XiHHG experiment. To understand the observed (and not observed) behaviour of the absorption spectra we are currently performing simulations.
References and relevant links
[1] Schafer et al., Phys. Rev. Lett. 92, 023003 (2004).
[2] Gademann et al. New Journal of Physics 13, 033002 (2011).
[3] Gallmann et al., Molecular Physics 111, 2243 (2013).
[4] Chini et al., Scientific Reports 3 (2013).
Introduction
One of the aims of the current research is the study of ultrafast dynamics in the few-femtosecond/attosecond regime in solid, liquid and gas targets. The main approach that has been followed and implemented until now is Attosecond Transient Absorption (ATA) at the extreme ultra-violet (XUV). However, in some targets (like liquids or solid surfaces) the static absorption is so high that no photons reach the detectorand also in other cases the resolution of our XUV detector may be too low to resolve high energy photons.
On the other hand, transient absorption by core electrons in carbon-based molecules may produce high energy Auger electrons that give additional information on the underlying dynamics besides the absorbed photon spectrum. The goal of this project is to develop an electron spectrometer for our beamline, allowing us to look, not only at the absorption of the harmonics, but also at the spectrum of the ionized electrons.
Experiment
The Magnetic Bottle Electron Spectrometer (MBES) [1-3] is a time-of-flight spectrometer with some additional magnetic fields generated to steer the electrons emitted in a 2π/4π solid angle towards the detector without changing their kinetic energy.This capability yields to a vast improvementin the collection efficiency and allowing for the detection of even small numbers of electrons.
The current design of our MBES (Figure 1 - left) consists of a permanent magnet and a coil, to create the required magnetic fields, and some retarding electrodes to lower the speed of the electrons. Some initial simulations on the electron trajectories on this scheme have been performed using the commercial software SIMION.
- Magnetic field: the simulations show how a magnetic field varying smoothly from values clos e to 1 T near the interaction region to approximately 1 mT in the drifting tube (Figure 1, right) is created.
- Trajectories: the process of steering the electrons was observed and the parameters tweaked in order t o collect a 2π soli d a ngle of the emitted electrons (Figure 2, top).
- Electron spectra:It was determined that it could be possible to reach resolutions up to 0.6 eV for 60 eV e lectrons and for 300 eV aft er applying a retardation potential (Figure 2, bottom).
Even though the development of this MBES is still in an early phase, these initial results show that it may fulfil our needs in terms of energy resolution around the energies we are interested to study (Photon energies from 30 eV to 200-300 eV that would produce similar electron energies). The next steps will consist on making a technical design and performing the corresponding more detailed modelling for the magnetic fields and trajectories, as well as the interaction chamber to accommodate the different targets.
Current researchers
A. Sanchez-Gonzalez and Z. Diveki
Reference and relevant links
[1]: P. Kruit et al., J. Phys. E: Sci. Instrum. 16, 313 (1983)
[2]: A.M.Rijs et al., J. Electron Sprectroc. 112, 151 (2000)
[3]: M. Mucke et al., Rev. Sci. Instrum. 83, 063106 (2012)
Introduction
The interaction of ultra-short laser pulses with atoms and molecules in the gas phase has led, through High Harmonic Generation (HHG), to the ability to spectroscopically measure ultrafast effects, such as femtosecond-scale nuclear motion and sub-femtosecond scale multi-electron dynamics.
Over the last few years it has been experimentally proven that the process of HHG is also possible in bulk dielectric media [1,2]. This leads to the question of whether spectroscopic measurements that have proved so successful in gas phase media could also be employed in dielectrics. The currently dominant hypothesis is that HHG in dielectrics is due to the current formed by electron motion in the conduction band, in particular due to Bragg reflections at the edge of the Brillouin zone.
Theory
We have shown that simple classical models of intraband current can be extended to a semiclassical model by deriving a method for the calculation of sub-cycle transition dynamics between energy band within the dielectric [3]. Using this model we predicted that for certain laser parameters the electron currents in the conduction band will destructively interfere, leading to a loss of harmonic signal [3].
We have now started collaboration with Vladislav Yakovlev (Max-Planck-Institut fur Quantenoptik, Garching) to develop methods for solving the Time Dependent Schrodinger Equation (TDSE) in dielectrics, and include propagation of the laser field through such media. This work is in preparation for publica tion.
Current researchers
Peter G. Hawkins and Misha Yu Ivanov.
References and relevant links
[1] S. Ghimire, et al., Nat phys. 7, 138 (2011).
[2] O. Schubert et al., Nat Photon (2014).
[3] P.G. Hawkins and M.Y. Ivanov, Physical Review A 87 (6), 063842 (2013).