Colloquia and Seminars
Connectomics Facilities
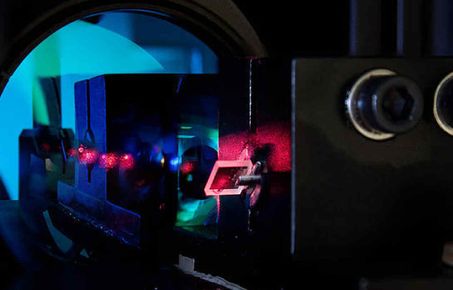
TissueCyte 1000 multiphoton tissue scanner
Details will follow shortly.
More information
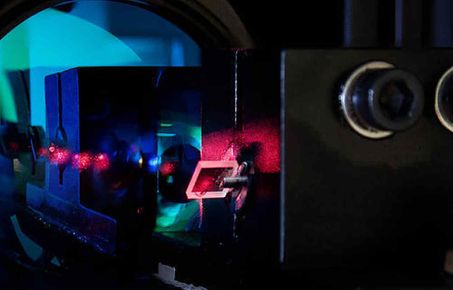
Ti:Sapphire laser
Details will follow shortly.
More information
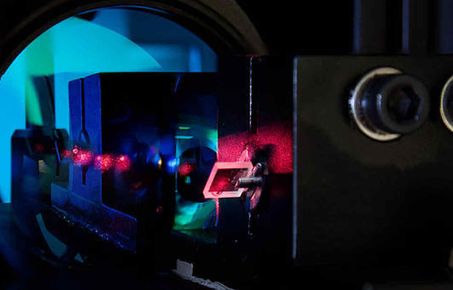
Automated organ-sectioning microtome
Details will follow shortly.
More information
CDT staff
Photo | Name | Role |
---|---|---|
![]() |
Simon Schultz | Centre/CDT Director & Director of MRes Neurotechnology Programme |
![]() |
Kate Hobson | Centre/CDT Administrator |
![]() |
Claudia Clopath | CDT Cohort Mentor - cohort 1 |
![]() |
Dan Goodman | CDT Cohort Mentor - cohort 2 |
Key Contacts
Internships
How to apply
General content colour blocks how to apply CDT
Apply for a funded project
- Check that you meet our eligibility criteria and entry requirements below
- Visit our projects page for details of available projects
- Visit our frequently asked questions page for tips on how to apply (including course codes)
- Submit an online application via the Imperial College online system
- Complete the project selection form to confirm which project(s) you are applying for (link available after projects are advertised)
Apply as a self-funded candidate
- Check that you meet the eligibility criteria and entry requirements below
- Visit our supervisors page for a list of potential supervisors
- Contact your preferred supervisor(s) directly to discuss opportunities and develop a project. All students must have two (or more) supervisors with complementary backgrounds, but the second supervisor can be chosen later.
- Once you have support from your main supervisor, submit an online application via the Imperial College online system (normally by 31st March).
You should choose the "Postgraduate Research" option, then "Combined Masters and PhD Application (1+3 Degrees)". Select Programme Title: "Neurotechnology (1plus3) (MRes 1YFT)|H6NT|1|SK|FT|CN".
Internships
2 column colour block how to apply

The 4-year CDT Programme
The CDT Neurotechnology Programme will not be admitting students for 2019/20. Applicants may wish to apply instead for the one-year MRes in Neurotechnology hosted by the Bioengineering Department. PhD opportunities may also be available through the Home departments of CDT supervisors. Please contact the relevant departments for more information.
APPLICATION DETAILS
MRes projects
Some examples of potential projects are listed below. Please contact your proposed supervisor BEFORE MAKING YOUR APPLICATION, to discuss any of these, or an alternative project.
Supervisors | Project |
---|---|
James Choi (Bioengineering) Magdalena Sastre (Medicine) |
Drug Delivery Across the Blood-Brain Barrier using Therapeutic Acoustic Wavelets Neurological diseases are among the most difficult diseases to treat, because drugs cannot enter the brain, because cerebral capillaries are lined by a blood-brain barrier (BBB). |
Vincenzo De Paola (Brain Sciences) Anil Bharath (Bioengineering) |
Ultrafast phenotyping of optically cleared human cortical tissue grafts with lightsheet microscopy and automated image analysis Model organisms are commonly used for studies of brain physiology and pathogenesis, but the extent to which the principles uncovered can be translated to humans remains unclear. Recently, we established a new approach to study human cortical synaptic networks using transplanted donor-derived cells and intravital longitudinal imaging and discovered new cellular and synaptic phenotypes in Down syndrome (Real et al 2018). 1) Apply ScaleS (Hama et al 2015), a reagent which renders brain tissue transparent within hours, to optically clear human cortical tissue grafts; These tools will likely accelerate our understanding of how the complexity of the human neocortex is perturbed in several neuropsychiatric conditions. |
Dario Farina (Bioengineering) Angela Kedgley (Bioengineering) |
Model-based estimation of upper limb joint kinematics and kinetics using surface electromyography Electromyography (EMG) is one of the few methods that provide a window on muscle activity and hence muscle force production during functional movements. As one of its applications, research in myoelectric control has produced active prosthetic devices with multiple degrees-of-freedom (DOFs), paralleled by EMG-based control strategies. |
Juan Álvaro Gallego (Bioengineering) Claudia Clopath (Bioengineering) |
Mapping the geometry of the “neural manifold” across the primate sensorimotor system Recent studies of neural population activity during behaviour have consistently uncovered that it is dominated by a handful of —around ten— neural covariation patterns (Gallego et al., 2017; Shenoy et al., 2013). These patterns span a neural manifold, a low-dimensional surface in the high-dimensional space defined by the activity of all recorded neurons. We refer to the neural population activity within the manifold as latent dynamics. Remarkably, studying the neural manifold and its associated latent dynamics has helped advance our understanding of how the brain controls behaviour, providing insight into questions that had remained elusive when studying single neuron activity. The “manifold approach” has also helped made brain-computer interfaces (BCI) more robust (Gallego, Perich et al., 2020; Pandarinath et al., 2018). Yet, there are many open questions regarding the geometrical properties of the neural manifold, with important implications both in basic neuroscience and BCIs. The goal of this project is to perform the first systematic comparison of manifold properties across the main areas of the sensorimotor system: premotor cortex, primary motor cortex, and primary sensory cortex. Such comparison will be carried out in a dataset with simultaneous recordings of around a hundred neurons in each of these areas, obtained as monkeys performed the same reaching task —the dataset from (Gallego, Perich et al., 2020). To assess the geometry of the neural manifold, we will fit the neural data with a broad range of manifold models that incorporate different assumptions about the data: from principal component analysis, and factor analysis, to isomap, and autoencoder neural networks. We will then explore the ability to predict the monkey’s behaviour from each of these different manifolds as we vary their assumed dimensionality. We expect this comparative study to inform about the difference in computations performed across these areas during a given behaviour, and on how to improve BCI design. Dr. Gallego will bring to this project expertise in systems neuroscience, and neural data analysis, in particular focusing on the application of neural manifold approaches. Prof. Clopath is a leading expert in computational neuroscience and the application of mathematical methods and modelling tools to understand neural computations. This project would suit a student with good Python programming skills and a strong mathematical foundation (from electrical or biomedical engineering, physics or mathematics) and a strong desire to learn neuroscience. References J.A. Gallego, M.G. Perich, L.E. Miller, S.A. Solla. Neural manifolds for the control of movement. Neuron 94(5):978–84, 2017 |
Gregory Scott, Ines Violante | Classification of EEG responses to multi-dimensional transcranial electrical stimulation |
Previous projects (for information only)
CDT Neurotechnology projects
Projects available for 2024-25
Academic staff contact details
Supervisor | Title | Description |
Professor Anil Bharath | Uncovering the neural code of DRL agents | Neuroscience has evolved exquisite tools to probe the behaviour of neurons in biology. Yet, very few of these tools are applied to decipher the encoding of deep neural networks. This particularly true in the field of deep reinforcement learning (DRL), where layered artificial neural networks learn mappings from observations to policies, or control signals. In this project, we build on prior work in agents trained to perform visuo-motor control, such as guiding a robot arm towards a target. The aim is to answer specific questions on parameter distributions and activation statistics, comparing these as training algorithms are altered, or environments perturbed. |
Professor Anil Bharath | Neural Architectures for Predicting the Behaviour of Dynamical Systems | Fuelled by artificial neural architectures and backpropagation, data-driven approaches now dominate the engineering of systems for pattern recognition. However, the predictive modelling of the behaviour of complex dynamical systems - such as those governed by systems of coupled differential equations - remains challenging in two key ways: (i) long term prediction and (ii) out-of-distribution prediction. Recent progress in disentangled representations (Fotiadis et al, 2021) has nudged the field forward, but it is now time to return to the underlying neural architectures, seeking those that are better suited to the intrinsic dynamics implicit in a system of equations. We seek to explore different approaches to this problem, including Siamese network structures, progressive network growth or, perhaps, neurons which incorporate some form of plasticity. |
Professor Anil Bharath | Learning from Mostly Unlabelled Data | Although data-driven machine learning is now being used to build components of AI systems for medical diagnostics, there is the need to find ways of being able to learn representations that are well suited to new forms of imaging data for which ground truth does not exist. This problem is not fully addressed in the AI field, where it is often assumed that data are readily available with ground truth, and that the (data, label) tuple can be made sufficient. But as new ways of imaging or measurement become available, the "data" part of that tuple can be subtly or radically different. Subtle differences can probably be handled by transfer learning, but data that is radically different requires new approaches to learning good deep representations. This project will investigate new approaches to self or unsupervised learning that do not rely on ground truth labels and can be applied to different tasks. |
Professor Anil Bharath | Learning from Mostly Unlabelled Data | Although data-driven machine learning is now being used to build components of AI systems for medical diagnostics, there is the need to find ways of being able to learn representations that are well suited to new forms of imaging data for which ground truth does not exist. This problem is not fully addressed in the AI field, where it is often assumed that data are readily available with ground truth, and that the (data, label) tuple can be made sufficient. But as new ways of imaging or measurement become available, the "data" part of that tuple can be subtly or radically different. Subtle differences can probably be handled by transfer learning, but radical ones require new approaches to learning, such as **contrastive learning**. This project will investigate contrastive learning in the context of medical imaging data from a variety of sources. |
Professor Anil Bharath | Predictive Coding: A Strategy for Representation Learning? | Predictive coding as a technique that uses local learning rules to discover deep representations. |
Professor Chiu Fan Lee | Deep learning and renormalisation group | Deep learning is a type of machine learning algorithms that has been applied to diverse problems in the past decade. A surprising connection between deep learning and renormalization group, a conceptual framework originated from physics in the 70s, has recently been uncovered. This project will study this connection mathematical, and illustrate the relationship concretely using numerical methods. |
Dr David Labonte | 3D shape analysis via flow fields | Any two objects may differ in size and in shape. Size differences are typically obvious, but shape differences can be subtle, and challenging to quantify, because typical methods involve 2D measures such as characteristic lengths. Recent advances in computational methods have enabled detailed quantitative analyses on complex 3D shapes. In this project, you will learn and deploy one approach, based on a shape atlas. This approach is applicable to any set of 3D objects, and thus has substantial generality.Generating a shape atlas requires to estimate an anatomical model (i.e. template) as the mean of a set of input shapes. Subsequently, shape variation is quantified via the calculation of the deformation required to mold this mean shape onto a shape representing an individual from the population of relevant shapes. Mathematically, this approach represents deformation between shapes as the diffeomorphic transformation of flow fields. By means of a gradient descent optimisation scheme, the method is able to produce a statistical atlas of the population of shapes, so allowing both quantitative and detailed visual comparison of shape differences across objects.You will use this approach to investigate differences in shape across workers of a colony formed by social insects - leaf-cutter ants. Workers of this important pest species differ in weight by more than two orders of magnitude, and it is commonly speculated that this size-differences goes hand in hand with a task specialisation, leading to the definition of "worker castes". However, it remains entirely unclear whether such shape differences exist. The required workflow is established in the laboratory, and µCT scans of ant workers of different sizes are available. Your main task will be to process these scans using CT-segmentation software and Blender, to then deploy the developed workflow, and produce the shape Atlas, which will enable you to answer if ant workers of different castes differ only in size or also in shape. Recommended literature:Toussaint, N., Redhead, Y., Vidal-GarcÃa, M., Lo Vercio, L., Liu, W., Fisher, E. M., ... & Green, J. B. (2021). A landmark-free morphometrics pipeline for high-resolution phenotyping: application to a mouse model of Down syndrome. Development, 148(18), dev188631. |
Professor Holger Krapp | Fly-robot interface - neuronal control of biohybrid system | Neurobiological research on the integration of signals obtained from different sensor systems is often limited by the fact that the experimental animals are stationary, i.e. they are fixed to a holder within an experimental setup. The reason for this is that stable electrophysiological recordings from nerve cells are only possible if there is no relative movement between the recording electrode and the nerve cell. While this approach has successfully been used to discover some general principles of sensory information processing, it has two fundamental limitations: (i) under natural conditions the animal simultaneously receives input from virtually all its sensor systems, and (ii) together with the motor commands it generates efference copies (forward models) which may modify the way in which sensor signals are being processed. To approximate more realistic conditions when monitoring neuronal activities in the fly nervous system the animal will be mounted on a robot that is free to move in the laboratory environment. Signals of motion- sensitive interneurons will be recorded and used to control the steering of the robot, establishing a closed-loop fly-robot interface (FRI). This configuration will allow the experimenter to selectively disable individual sensor systems and assess their respective impact on multisensory integration in the fly nervous system. The project includes the refinement of miniaturized electrophysiological equipment, e.g. amplifier circuits, that can be mounted on a the robot to record neural signals of identified optic flow-processing interneurons. The recorded signals will be filtered and converted into motor commands for robot steering. The resulting feed-back system will be set up in a way that prevents the robot from colliding with any obstacles in its immediate environment while the trajectory of the FRI and the neuronal signals are recorded for further data analysis. |
Professor Holger Krapp | Electrophysiological characterization of optic flow processing interneurons in flying insects. | The visual system in many animals and humans contributes to state change estimations by analysing panoramic retinal image shifts known as optic flow. Earlier studies in blowflies have revealed the underlying neuronal mechanism which is believed to complement state change estimation based on mechanosensory/inertial systems. This project aims to support the idea that optic flow processing in the visual system of flying insects is tuned to control species-specific natural modes of motion which are determined by the animal's flight dynamics. Experimental evidence in support for this mode sensing hypothesis requires a comparative study of the receptive field properties of motion sensitive interneurons in the visual systems of dipteran flies other than blowflies as well as species that belong to other orders of flying insects. Hoverflies, which show distinctly different flight patterns than blowflies and perform differently in behavioural gaze stabilization experiments, would be an ideal candidate species. Preliminary experiments have shown that hoverflies, too, employ motion sensitive interneurons in their visual system. But only a few of those interneurons have been studied so far. This project requires the dissection of flying insects for extracellular recordings from motion sensitive interneurons during visual stimulation. The neuronal responses will be analysed using customized MATLAB/Python programmes which reveal the cell's receptive field organization. From the electrophysiological results the preference of individually identifiable interneurons for specific self-motion components (state changes) will be derived. The principles underlying state change estimates in flying insects will inform the design of energy-efficient flight control architectures for autonomous aerial systems. |
Professor Holger Krapp | 3d head rotations in blowflies | This desk-based project aims to retrieve fly 3D head rotations from 2D high speed videography data. By combining image analysis methods with 3D model fitting techniques, it studies the correlation of head roll, pitch, and yaw rotations induced by visual and inertial stimuli. The objective is to understand the functional organization and control of the fly neck motor system that provides the animal with a stabilized visual input based on compensatory head movements. The results are relevant for the development of image stabilization systems mounted on small autonomous robotic platforms. |
Professor Holger Krapp | Neuronal integration of polarised light and motion vision in flies and butterflies | Many animals, including flies and butterflies, exploit patterns of linearly polarized light to guide tasks as diverse as navigation, water seeking, mate recognition, communication, and host detection. Polarized light cues in natural scenes follow distinct spatiotemporal patterns, and visual systems have consequently evolved to process polarization cues within this dynamic context. Whilst polarization cues for communication and host detection are also thought to be processed as structured spatiotemporal signals, the mechanisms by which polarization information is integrated with object detection and motion vision pathways in these contexts is less well understood. This project aims to measure neuronal responses of motion sensitive interneurons to moving patterns of polarised light in flies and butterflies. The project will involve mounting and dissecting insects for extracellular electrophysiology, collecting wild flies and butterflies during the summer, and data analysis in Matlab. Programming experience is useful but can be learned as part of the project. |
Professor Holger Krapp | Electrophysiological characterization of forward model adaptation in walking flies | A stable and accurate perception of the world ultimately requires the distinction between sensory inputs that result from our intended self-motions, and those arising from external perturbations. Failure to tell apart these two scenarios would result in a futile cycle of our stabilization reflexes to counteract our intended movements. Recent neurophysiological evidence suggested that flies anticipate the sensory consequences of their actions and apply so-called forward models to prevent stabilization reflexes from occurring in response to volitional movements. In theory, these forward models must adapt to account for variable neuronal delays and changes in the sensory environment. The project will test this hypothesis by measuring the electrophysiological responses of motion sensitive interneurons in the fly brain whilst the fly is walking in a closed-loop virtual reality environment with control over the latency of sensory feedback signals. This project will involve (i) the dissection of flies to access neuronal circuits supporting motion vision while the animal is walking on a spherical treadmill, (ii) record extracellular signals from motion-sensitive interneurons and (iii) the analysis of the treadmill walking traces and neuronal recordings using Matlab or Python. Programming experience is useful but can be learned as part of the project. |
Professor Holger Krapp | Closed-loop polarotaxis in flying insects | Many insects, including flies and butterflies, orient themselves relative to the pattern of polarised skylight. This is thought to underly a form of navigation or spatial orientation, termed polarotaxis. Previous results in the lab suggest that flies are more likely to orient to a polarised light pattern when they are free to rotate, rather than when tethered in place on a wing beat analyser that enables the measurement of the animal’s intended turns. This difference may arise due to the absence of visual feedback when tethered in place and can be tested by closing the loop such that the animal’s movement is fed back to update the polariser orientation. The aim of this project is to compare polarotaxis in tethered flying insects, including flies and butterflies, both under open- and closed-loop conditions. Methods will include (i) image analysis for pose estimation (including neural network training in Python using DeepLabCut), (ii) programming stepper motors using Arduino, and (iii) data analysis in Matlab or Python. Familiarity with programming is helpful but could be learned at the start of the project. |
Dr Jun Ishihara | Effect of cancer immunotherapy on mental health | "Recent research revealed that excess immune system activation causes mental illness. Cancer immunotherapy is a revolutionary invention to fight cancer through activating the immune system. Together, it is suggested that immunotherapy may cause mental illness, however the molecular mechanism between immunotherapy and mental illness remain unclear. Serotonin and histidine are crucial molecules for regulating mental condition. Parry Hashemi lab has developed a biosensor that can monitor the serotonin/histamine level in the brain in vivo. Jun Ishihara is an immuno-engineer, who can produce immunotherapy drugs in his laboratory. Jun can make immunotherapeutics both unmodified versions (which rapidly distribute to the whole body after injection; thus systemic therapy) and engineered versions (which stick to ECM proteins at the injection site; thus localised therapy). Our central hypothesis is that cancer immunotherapy causes mental illness through serotonin/histidine concentration changes and cytokine release, and this could be solved by a drug delivery system approach. Systemic cancer immunotherapy may cause mental health issues. Engineered cancer immunotherapy that can localise at the cancer would reduce the mental health issue.This research will shed a light on connection between immunotherapy and mental illness, and can propose a solution for immunotherapy-induced mental illness. Students can learn various aspects of immunotherapy and neurotechnology related techniques." |
Dr Majid Taghavi | Multimodal haptic device | Haptic stimulation is an innovative technology that leverages the sense of touch to convey information to users. Traditionally, this has been achieved through vibrations to deliver notifications. However, in this project, our aim is to develop a novel wearable technology capable of delivering a diverse range of subtle mechanical stimulations, including tapping and sliding, to the wearer. This device could be used for sending complex physical information to individuals with visual, hearing, or mobility impairments, providing cutaneous sensory re-education, or therapeutic guidance to the patient during an activity. |
Dr Majid Taghavi | Interactive robotic skin | Human can examine structures or areas for size, shape, consistency, texture, and other characteristics simply by moving their fingers and palms over the surfaces. Dexterous movement, flexible and soft architecture, and a variety of sensing capabilities on the skin enable this palpation. In this project, we aim to advance this concept by developing a cutting-edge soft robotic material. This material will incorporate embedded electroactive artificial muscles and tactile sensors, creating an interactive composite working like a robotic skin. By employing this innovative technology, it will be possible to scan the body and accurately detect variations in stiffness, opening up new possibilities for medical diagnostics and interventions |
Dr Majid Taghavi | Soft-rigid hybrid robotic material | Soft robotics built from highly compliant materials suggests safe and adaptable technologies for many applications including human-machine interactions (e.g. surgical robots, implants, and wearables). In practical uses, it is usually needed to integrate dissimilar materials, such as soft with rigid and electrodes with non-conductors, in the architecture of soft robots for applying mechanical support and electrical connections. Inspired by nature, where dissimilar materials are found with progressive compositional changing (e.g. muscle-tendon-bone), in this research we will develop a novel robotic material with a smooth transition from soft to rigid, embedding a unique capability of interacting with the environment. This soft-rigid hybrid muscle will benefit from a reliable connection to rigid bodies, withstanding diverse mechanical deformation and robust electrical connections. |
Dr Majid Taghavi | Variable stiffness soft robotic material | Variable stiffness materials provide a core technology for wearables and assistive robotics. They are a class of smart materials with the capability of stiffness change under external stimulation. Within this project, we will develop different types of electroactive ionic elastomers for variable stiffness applications. The intrinsic mechanical properties of the ionic elastomer and their response to the applied electric field will also be characterised. We will then design a proof of concept robotic component to demonstrate its use in wearables and implants. |
Dr Majid Taghavi | Nylon thread artificial muscle | Coiled polymer artificial muscles offer a new type of thermo-active soft actuation technology with high potential to be used in wearables and assistive robotics. A twisted and coiled sewing thread such as silver-coated Nylon can generate large stroke and high-power density when actuated by ohmic heating. They however suffer from the lack of high bandwidth controllability because of the slow cooling process. In this project, we aim to tackle this challenge by implementing a multi-physical approach and introducing intelligent structures to extend their applicability in interactive textile technologies. |
Dr Majid Taghavi | Social soft robot | Social robots are robots that interact and communicate with humans, usually to provide some kind of social or service function. They are becoming increasingly common in settings such as healthcare, education, and the home. In this project, you will develop a new type of social robot that is made of soft material and can change its shape and characteristics using soft actuators to communicate with people. For example, the robot could change its shape to communicate different emotions, such as smiles, anger, or fear. |
Professor Rylie Green | Living Bionics: Stimulation to drive neural network development | Electrical stimulation has been demonstrated to induce directional neurite growth in various cell types, both human and non-human using biphasic stimulation. This research project aims to evaluate a range of sinusoid stimulation frequencies to drive activity, growth and release of neurotransmitters of developing neurons using a cell stimulation rig made in house. |
Professor Rylie Green | Spinal cord bridge | Nerve regeneration in an injured spinal cord is often restricted. One possible reason may be the lack of topographical signals from the material constructs to provide contact guidance to invading cells or re-growing axons. This research project aims to evaluate randomly oriented or aligned collagen fibers coated on cuff electrodes to study device topographical effects on astrocyte behavior and neurite outgrowth respectively, using electrical regimes. |
Professor Rylie Green | Biofunctionalising electrodes through conductive hydrogel coatings | Peripheral nerves cuffs can have there biointegration improved through the use of conductive hydrogel coatings. One of our long-term goals is to improve the long term performance of these electrodes. This projects will investigate reducing inflammation at the site of implantation by the incorporation and release of anti-inflammatory mediators from the hydrogel coatings. Different mediators will be investigated along with different release methods and how these impact the performance of the cuff electrodes. |
Professor Rylie Green | A bioelectronic implant for cancer treatment | This project revolves around aiding in the development of a device for the selective delivery of chemotherapy directly to the site of non-operable brain tumors (glioblastoma multiforme). This device consists of a conductive polymer-based material that can used as an electrically controlled drug delivery system. The goal of this project is to evaluate the drug release profiles for multiple different molecules that are analogs to those commonly used in chemotherapy. Parameters such as molecule size, charge, and stability will be investigated. Characterization of the drug release profiles will be accomplished through chemical, electrochemical, and spectroscopic techniques. |
Professor Rylie Green | In ear monitoring of EEG | This project is a funded collaboration with EEE and international partners. It seeks to deliver accurate EEG and other biosignal information through eletronics mounted in an ear bud. The project will focus on the design and characterisation of dry wearable electrodes and the identification of biosignals through processing. The areas of focus will span biomaterials, electronics, signal processing and neural netoworks. |
Dr Reiko Tanaka | Systems biology approach for mechanistic understanding of paediatric asthma exacerbations | Asthma is the most common chronic disease of childhood, affecting up to 10% of children in Westernised societies and 200,000,000 individuals worldwide. Many factors indicate the importance of the microbiome in asthma. Asthma is rare in rural societies, and its prevalence has been increasing markedly in the developing world as populations become urbanised. Exacerbations of asthma are often precipitated by otherwise trivial viral infections. Our studies have shown that the normal human airways contain a characteristic microbiome that is altered in children and adults with the illness. Asthmatic airways contain an excess of pathogens (which may damage the airways) and also lack particular commensal species that may be necessary for normal airway functions. This project will take a systems biology approach, by combining experiments with primary bronchial epithelial cells, in silico modelling, and clinical data analysis, to elucidate the effects of the airway bacterial microbiome in asthma, and the role of epithelium barrier integrity in disease initiation and control. We already have a preliminary mathematical model that will be used to quantify the dynamic interactions among pathogen, commensals at the airway surface, the airway barrier and the immune system, preliminary data from in vitro experiments, and clinical data to be analysed. The student(s) will conduct several computational methods to identify the model structures and model parameters. |
Dr Reiko Tanaka | Systems biology approach for cancer immunotherapy: Dynamical mechanisms to turn hot tumours into cold tumours | Immunotherapy is a promising treatment for cancers. Several immunotherapy treatments have already achieved clinical success. For example, checkpoint inhibitors (CPIs) targeting PD1 and CTLA4 achieved up to 11% of cure rates in advanced melanoma. However, its treatment response rates remain low for a majority of cancers. This project aims to apply a systems biology approach to increase the efficacy of CPI therapies. CPI therapy achieves cancer regression by preventing exhaustion of T cells that pre-exists in the tumours but does not exhibit strong antitumour immunity if tumours have few immune cells. One way to improve the CPI efficacy is to convert immune-excluded cold tumours into immune-infiltrated hot tumours, by enhancing infiltration of tumour-specific immune cells into the tumour tissue. Ishihara et al have recently engineered a tumour-homing anti-tumour cytokine IL-12 (Nat Biomed Eng 2020) that turns immunologically cold tumours into hot ones, and demonstrated a high antitumor efficacy of a combination therapy using IL-12 and CPI for cold tumors. However, the actual dynamical mechanisms by which IL-12 converts cold tumours to hot tumours and how IL-12 acts in combination with CPI therapy is still elusive. This project aims to investigate the cellular mechanism of immune cells infiltration during the combined therapy. The student will develop and analyse a computational model of immune cells infiltration based on the results from in vitro and in vivo experiments, to suggest the best timing of adding CPI theraty to achieve the most anti-tumour efficacy. |
Dr Reiko Tanaka | Learning from noisy labels by EczemaNet | Eczema is the most common form of skin disease. The eczema severity is currently assessed by trained clinical staff. However, the subjective nature of assessing these disease signs could be a source of significant inter and intra-observer variability. Our group recently developed EczemaNet, a prototype novel computer vision pipeline for automated evaluation of eczema severity from camera images (Pan, Hurault et al. 2020). EczemaNet uses a CNN that first detects areas of AD from camera images and then makes probabilistic predictions of the severity of seven clinical signs of AD. EczemaNet was trained using the camera images with eczema severity scores provided by trained clinical staff. However, the severity scores may have inter-rater variability, resulting in noisy labels. This project aims to further improve EczemaNet by achieving a better generalisation capability in the presence of noisy labels. The student is expected to review, implement, and validate the image analysis pipeline using off-the-shelf deep-learning and image analysis techniques and software packages to improve the prediction accuracy in the presence of noisy labels. |
Dr Reiko Tanaka | Automatic quantification of fungal burdens in histology images using deep neural networks | Invasive fungal infections are commonly treated by antifungal drugs, which are under the growing threat of antifungal resistance. New antifungal drugs that overcome antifungal resistance are being sought actively. Effectiveness of new antifungal drugs is often tested in vitro by comparing fungal growth rates estimated from time-course optical density (OD) data of fungal burden in different treatment conditions. However, OD is an indirect measure of fungal burden, with potential bias in the evaluated growth rates and artefacts in the data such as multiple scattering. Various methods to improve bacterial growth rate estimation from OD have been proposed and subsequently been made available to the wider bacterial community. However, these methods require converting OD to cell counts in a process called calibration, which is not routinely available for fungi. |
Dr Sophie Morse | Do immune cells enter the brain when delivering drugs across the blood-brain barrier with focused ultrasound? | Focused ultrasound and microbubbles is the only technique that can non-invasively, locally and temporarily open the blood-brain barrier to allow drugs into the brain. The way this technology works is by first injecting clinically-approved microbubbles and drugs into the bloodstream. When the microbubbles reach the area where the ultrasound is targeted in the brain, these bubbles oscillate, mechanically stimulating the blood vessels, allowing the barrier to open so that drugs can get reach the brain. This technology has generated a lot of excitement as the blood-brain barrier currently prevents over 98% of drugs from entering the brain. Therefore, this technique can allow drugs that have previously failed clinical trials due to this barrier, to be tested again. But do immune cells also infiltrate from the blood into the brain, together with the drug, while the blood-brain barrier is open? In some situations immune cell infiltration would want to be avoided, for safety reasons. However, in other cases, such as when treating brain tumours, the presence of peripheral immune cells inside tumours could really help. Our own immune cells are actually best suited at tackling the complex heterogeneity of brain tumours. In this project, you will explore with sectioning, staining and fluorescence microscopy whether different types of immune cells are extravasating into the brain when the blood-brain barrier is opened with focused ultrasound. Ultimately, the aim is to find out with which ultrasound parameters you would get immune cells entering the brain, to either choose parameters for a safer drug delivery profile without immune cell presence, or to harness the power of our own immune cells to treat brain tumours. |
Dr Sophie Morse | Can focused ultrasound stimulate the activity of the brains innate immune cells? | Microglia are the innate immune cells of our brain. They actively survey the brain and clear away toxins and pathogens. The ability to temporarily stimulate microglia has the potential to help treat brain diseases, such as brain tumours, Alzheimers disease and Parkinsons disease. For example, stimulating microglia can help clear away amyloid-beta plaques that accumulate in Alzheimers disease brains. Focused ultrasound is a non-invasive technique that has been used to stimulate neurons. However, we have discovered that the activity of microglia can also be modulated depending on the way ultrasound is emitted. In this project, the level of microglia activation will be assessed for a variety of different ultrasound exposure parameters. Staining for various markers of microglia and their activation will be used to visualise (with microscopy) changes in their morphology and activation level. This project will lead to key advances in the unexplored territory of how the activity of the innate immune cells in our brain can be non-invasively controlled with ultrasound. |
Dr Sophie Morse | Can focused ultrasound stimulate astrocytes in our brain? | Astrocytes, together with endothelial cells, are the gatekeepers of the brains main security system “ the blood-brain barrier. They control which substances enter and exit the brain, and keep harmful substances out. Astrocytes also regulate blood flow to transport nutrients to neurons depending on their energy needs. These cells are at the forefront of our thought processes: they regulate our synapses, and recycle and secrete neurotransmitters. The ability to temporarily stimulate astrocytes has the potential to help treat brain diseases, such as Alzheimers disease and Parkinsons disease. Focused ultrasound is a non-invasive and targeted technique that has been used to stimulate neurons. However, we have discovered that the activity of astrocytes can also be modulated depending on the way ultrasound is emitted. In this project, the level of astrocyte activation will be assessed for a variety of different ultrasound exposure parameters. Staining for various markers of astrocytes and their activation will be used to visualise (with microscopy) changes in their morphology and activation level. This project will lead to key advances in the unexplored territory of how the activity of the brains gatekeepers can be non-invasively modulated with ultrasound to treat brain diseases. |
Dr Sophie Morse | Can focused ultrasound delay Alzheimer's disease? | Focused ultrasound is a technology that has very recently shown to restore cognitive function in Alzheimer's disease patients. This is a non-invasive technology that can be focused onto specific regions of the brain. One theory is that this technology can restore cognition by stimulating the innate immune cells of the brain as well as neuronal function and health. In this project you will explore 1) whether this same technology can be used to delay Alzheimer's as well as restore cognition and 2) delve into exploring the mechanisms behind why these effects are observed. This will involve working with mouse brain tissue, sectioning, imaging, staining and fluorescence microscopy. |
Dr Sophie Morse | Can focused ultrasound delay brain ageing? | Focused ultrasound is a technology that has very recently shown to restore cognitive function in Alzheimer's disease mice and patients. This is a non-invasive technology that can be focused onto specific regions of the brain. One theory is that this technology can restore cognition by stimulating the innate immune cells of the brain as well as neuronal function and health. In this project you will explore 1) whether this same technology can be used to delay age-related cognitive decline, as well as restore cognition in Alzheimer's disease, and 2) delve into exploring the mechanisms behind why these effects are observed. This will involve working with mouse brain tissue, sectioning, imaging, staining and fluorescence microscopy. |
Projects
Cortexica are leaders in AI, Machine Learning and Computer Vision for image and video analysis. Focused on creating and turning cutting-edge AI and machine learning research into real world solutions that make businesses commercially stronger. Starting life from the Bioengineering Department at Imperial College London, Cortexica originated from a research project to reverse-engineer the human visual cortex, and now has its own team of PhD researchers and engineers that form the back bone of its video centre of excellence. |
|
![]() |
GripAble, formed by researchers at Imperial College London and clinicians at Imperial College Healthcare NHS Trust, is a digital assessment and training platform supporting individuals undergoing rehabilitation for both neurological and musculoskeletal conditions. With a focus on hand and arm function, rehabilitation programmes are delivered through interactive mobile technologies that have been designed to motivate, track progress and provide real-time biofeedback. |
![]() |
Spun-out from the research of Centre member Professor Rylie Green, Polymer Bionics produces novel, conductive, non-metallic materials for electrodes with improved body compatibility enabling safer, longer lasting bionic devices. |
![]() |
SERG Technologies is an Imperial College deep tech spin-out company, with a mission to revolutionise the way humans interact with technology by re-inventing human to machine interface and develop anthropocentric solutions in the healthcare field that will improve the quality of life for millions of people. The company was founded by Ravi Vaidyanathan, Samuel Wilson, Alex Lewis and Christos Kapatos. SERG is working closely with NHS hospitals and leading UK private clinics as well as with market-leading companies in the prosthetics and orthotics, medical and homecare fields, creating partnerships, raising awareness and promoting its technology. |
The Centre for Neurotechnology and its members are involved with a number of large-scale collaborative research projects which incorporate multidisciplinary research groups from within and outside of Imperial College. In addition we have a number of official partners and affiliates of the Centre and CDT.
Imperial Network of Excellence in Neuromuscular Rehabilitation Technology
The Neuromuscular Rehabilitation Technology Network (NRTN) brings together biomedical scientists/clinicians and physical scientists/engineers to forge new collaborative ventures. The purpose of the network is to harness the strengths of Imperial expertise in convergent research across the departments and faculties through multidisciplinary and collaborative initiatives, delivering medical technology innovation that impacts fields of unmet healthcare need. The NRTN and Centre for Neurotechnology are closely aligned; many Centre members work in the area of reurorehabilitation and are also involved with the NRTN, and the Centre for Doctoral Training in Neurotechnology for Life and Health currently funds several studentships in the area of neurorehabilitation.
Neurotechnology for Memory Enhancement
Neural Engineering Transformative Technologies (NETT)
Neural Engineering brings together engineering, physics, neuroscience and mathematics to design and develop brain-computer interface systems, cognitive computers and neural prosthetics. Neural Engineering Transformative Technologies (NETT) is a Europe-wide consortium of 18 universities, research institutes and private companies who together will host 17 PhD students and 3 postdoctoral researchers over the next 4 years. NETT has been made possible by a €5.3M grant by the European Commission through its Marie Curie Initial Training Network scheme. Researchers from the Centre for Neurotechnology and NETT collaborate on a number of projects and the two groups organise a joint Winter School every two years.
The three-photon system comprises a Scientifica Hyperscope and a Satsuma 40W fiber laser with a Mango SP Optical Parametric Amplifier which has a tunable wavelength range of 650-920 nm and 1150-2500 nm. The system is ideal for imaging deep within scattering tissue in vivo and ex vivo.
Multi-photon microscopy has the advantage of deeper tissue penetration with reduced photobleaching compared to single-photon confocal microscopy. While two-photon microscopy allows structures or physiological activity to be imaged up to a depth of about 1 mm, the longer excitation wavelength in three-photon microscopy allows deeper imaging. Moreover, the light is scattered less resulting in greater image contrast in three-photon than in two-photon microscopy.
This equipment is available for use by any member of Imperial College.
To book, click here. For enquiries, please contact the Facility Manager, Ann Go.
Location: Bessemer B532D, South Kensington