Divert to blog, google scholar and OA
Topics
- Recent Highlights
- Functional molecular systems
- Coarse-grained modelling of DNA
- Thermodynamics of small systems
- Simulation tools and algorithms
- Biomolecular Engineering
We derive general thermodynamic bounds on information propagation in complex catalytic molecular templating networks. We thereby make rigorous a postulated link between stochastic thermodynamics and the crucial class of biochemical processes that determine cellular RNA and protein levels. In so doing, we make a fundamental discovery: information propagation in this context is limited by simple network properties. Moreover, optimal information processing involves a pseudo-equilibrium limit with negligible entropy production. These results shift the paradigm of molecular information processing, which has hitherto been assumed to require entropy production, and open up a new approach for engineering synthetic, low-cost information processing networks.
Backbone-assisted assembly processes -- such as protein folding -- allow the assembly of a large number of structures with high accuracy from only a small handful of fundamental building blocks. We aim to explore general principles underlying this phenomenon by studying variants of the temperature-1 abstract tile assembly model (aTAM). We consider the existence of finite sets of tile types that can deterministically assemble any shape producible by a given assembly model; we call such tile type sets universal assembly kits. Our first model, which we call the ``backboned aTAM", generates backbone-assisted assembly by forcing tiles to be be added to lattice positions neighbouring the immediately preceding tile, using a predetermined sequence of tile types. We demonstrate the existence of universal assembly kit for the backboned aTAM, and show that the existence of this set is maintained even under stringent restrictions to the rules of assembly. We hypothesise that the advantage of the backboned aTAM relative to the conventional aTAM -- which does not possess such a set -- is in part due to the specification of a sequence, and in part due to the geometric restrictions imposed by the backbone. To explore this intuition, we develop a second model call the ``sequenced aTAM". The sequenced aTAM uses a predetermined sequence of tiles, but does not constrain a tile to neighbour the immediately preceding tiles. We prove that this model has no universal assembly kit. The lack of such a kit is surprising, given that the number of tile sequences of length N scales faster than the number of producible shapes of size N for a sufficiently large -- but finite -- set of tiles.
Dec 2023: Exploiting bias in optimal finite-time copying protocols (submitted)
We study a finite-time cyclic copy protocol that creates persisting correlations between a memory and a data bit. The average work to copy the two states of the data bit consists of the mutual information created between the memory and data bit after copying, a cost due to the difference between the initial and final states of the memory bit, and a finite-time cost. At low copy speeds, the optimal initial distribution of the memory bit matches the bias in the expected outcome, set by the bias in the data bit and the copying accuracies. However, if both states of the data are copied with the same accuracy, then in the high-speed regime copying the unlikely data bit state becomes prohibitively costly with a biased memory; the optimal initial distribution is then pushed towards 50:50. Copying with unequal accuracies, at fixed copy-generated mutual information, yields an opposite yet more effective strategy. Here, the initial memory distribution becomes increasingly biased as the copy speed increases, drastically lowering the work and raising the maximum speed. This strategy is so effective that it induces a symmetry breaking transition for an unbiased data bit.
Nov 2023: Strong sequence dependence in RNA/DNA hybrid strand displacement kinetics (submitted)
Strand displacement reactions underlie dynamic nucleic acid nanotechnology. The kinetic and thermodynamic features of DNA-based displacement reactions are well understood and well predicted by current computational models. By contrast, understanding of RNA/DNA hybrid strand displacement kinetics is limited, restricting the design of increasingly complex RNA/DNA hybrid reaction networks with more tightly regulated dynamics. Given the importance of RNA as a diagnostic biomarker, and its critical role in intracellular processes, this shortfall is particularly limiting for the development of strand displacement-based therapeutics and diagnostics. Herein, we characterise 22 RNA/DNA hybrid strand displacement systems, systematically varying several common design parameters including toehold length and branch migration domain length. We observe the differences in stability between RNA-DNA hybrids and DNA-DNA duplexes have large effects on strand displacement rates, with rates for equivalent sequences differing by up to 3 orders of magnitude. Crucially, however, this effect is strongly sequence-dependent, with RNA invaders strongly favoured in a system with RNA strands of high purine content, and disfavoured in a system when the RNA strands have low purine content. These results lay the groundwork for more general design principles, allowing for creation of de novo reaction networks with novel complexity while maintaining predictable reaction kinetics.
Kinetic proofreading is used throughout natural systems to enhance the specificity of molecular recognition. At its most basic level, kinetic proofreading uses a supply of chemical fuel to drive a recognition interaction out of equilibrium, allowing a single free-energy difference between correct and incorrect targets to be exploited two or more times. Despite its importance in biology, there has been little effort to incorporate kinetic proofreading into synthetic systems in which molecular recognition is important, such as nucleic acid nanotechnology. In this letter, we introduce a DNA strand displacement-based kinetic proofreading motif, showing that the consumption of a DNA-based fuel can be used to enhance molecular recognition during a templated dimerization reaction. We then show that kinetic proofreading can enhance the specificity with which a probe discriminates single nucleotide mutations, both in terms of the initial rate with which the probe reacts and the long-time behaviour.
Information propagation by sequence-specific, template-catalyzed molecular assembly is the source of the biochemical complexity of living systems. Templating allows the production of thousands of sequence-defined proteins from only 20 distinct building blocks. By contrast, exploitation of this powerful chemical motif is rare in non-biological contexts, particularly in enzyme-free environments, where even the template-catalyzed formation of dimers is a significant challenge. The main obstacle is product inhibition: the tendency of products to bind to their templates more strongly than individual monomers, preventing the effective catalytic templating of longer polymers. Here we present a rationally designed enzyme-free system in which a DNA template catalyzes, with weak competitive product inhibition, the production of sequence-specific DNA dimers. We demonstrate the selective templating of 9 different dimers with high specificity and catalytic turnover. Most importantly, our mechanism demonstrates a rational design principle for engineering information propagation by molecular templating of longer polymers.
Dynamic DNA nanotechnology involves the use of DNA strands to create programmable reaction networks and nanodevices. The key reaction in dynamic DNA nanotechnology is the exchange of DNA strands between different molecular species, which is achieved through three‐way and four‐ way DNA exchange reactions. While both of these reactions have been widely used to build reaction circuits, the four‐way exchange reaction has traditionally been slower and less efficient than the three‐way reaction. In this paper, we describe a new mechanism to optimise the kinetics of the four‐ way DNA exchange reaction by adding bulges to the toeholds of the four‐way DNA complexes involved in the reaction. These bulges facilitate an alternative branch migration mechanism and destabilise the four‐way DNA junction, increasing the branch migration rate and unbinding rate of the four‐way exchange reaction, bringing it closer to the kinetics of the three‐way reaction. This new mechanism has the potential to expand the field of dynamic DNA nanotechnology by enabling efficient four‐way DNA exchange reactions for in vivo applications.
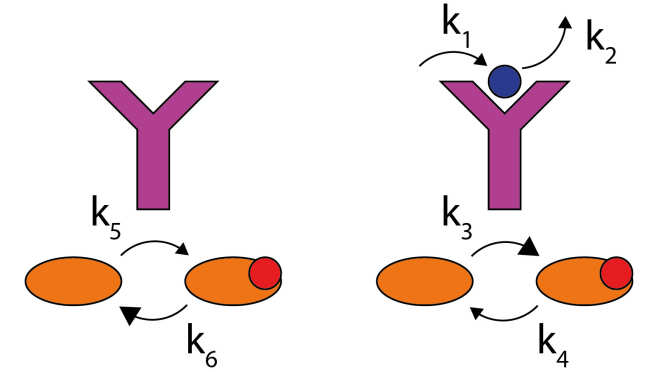
Biochemical networks within cells achieve remarkable functionality, including sensing, signalling, information-processing and replication. We aim to understand the fundamental physical principles that set the scope of this behaviour, allowing development of engineering principles for artificial analogs of these systems.
Since these systems of interest typically involve small numbers of molecules, randomness plays an important role. This work often touches on the deep connections between two bodies of work that deal explicitly with randomness: information theory, which describes the role of randomness in communication, and statistical mechanics, through which randomness is related to thermodynamics.
Recently, we've started to translate our basic understanding into the engineering of synthetic, nucleic acid-based analogs of these natural systems within our own lab here at Imperial. To get a taste, check out Javi's talk at FNANO2020, or a simplified discussion of his overall project.
Relevant Publications
- Plesa T, Dack A, Ouldridge TE, 2023 "Integral feedback in synthetic biology: Negative-equilibrium catastrophe", J. Math. Chem.
- Qureshi, B., Juritz, J., Poulton, J. M., Beersing-Vasquez, A., & Ouldridge, T. E. (2023). A universal method for analyzing copolymer growth. The Journal of Chemical Physics.
- JM Lawrence et al., 2022, Synthetic biology and bioelectrochemical tools for electrogenetic system engineering, Science Advances.
- Catala AC, Ouldridge TE, Stan GBV, Bae W, 2022, "Building an RNA-Based Toggle Switch Using Inhibitory RNA Aptamers", ACS Synthetic Biology.
- Juritz J, Poulton JM, Ouldridge TE, 2021, "Minimal mechanism for cyclic templating of length-controlled copolymers under isothermal conditions", The Journal of Chemical Physics.
- Bae W, Stan GBV, Ouldridge TE, 2021, "In situ generation of RNA complexes for synthetic molecular strand displacement circuits in autonomous systems", Nano Lett.
- Cabello-Garcia J, Bae W, Stan GBV, Ouldridge TE, 2021, "Handhold-mediated strand displacement: a nucleic acid-based mechanism for generating far-from-equilibrium assemblies through templated reactions", ACS Nano.
- Poulton JM, Ouldridge TE, 2021, "Edge-effects dominate copying thermodynamics for finite-length molecular oligomers", New J. Phys.
- Lankinen A, Mullor Ruiz I, Ouldridge TE, 2020, "Implementing Non-Equilibrium Networks with Active Circuits of Duplex Catalysts", 26th International Conference on DNA Computing and Molecular Programming (DNA 26).
- Plesa T, Stan G-BV, Ouldridge TE, Bae W, 2021, "Quasi-robust control of biochemical reaction networks via stochastic morphing", J. R. Soc. Interface.
- Deshpande A, Ouldridge TE, 2020, "Optimizing enzymatic catalysts for rapid turnover of substrates with low enzyme sequestration". Biological Cybernetics.
- Brittain RA, Jones NS, Ouldridge TE, 2019, Biochemical Szilard engines for memory-limited inference, New J. Phys.
- Poulton J, ten Wolde PR and Ouldridge TE, 2019, Non-equilibrium correlations in minimal dynamical models of polymer copying, PNAS.
- Ouldridge TE, Brittain RA, ten Wolde PR, 2018, The power of being explicit: demystifying work, heat, and free energy in the physics of computation, in "The Energetics of Computing in Life and Machines", SFI Press.
- Deshpande A, Ouldridge TE, 2017, High rates of fuel consumption are not required by insulating motifs to suppress retroactivity in biochemical circuits, Engineering Biology.
- Poole W, Ortiz-Muñoz A, Behera A, Jones NS, Ouldridge TE, Winfree E, Gopalkrishnan M, 2017, Chemical Boltzmann Machines, In DNA Computing and Molecular Programming (DNA 2017).
- Brittain RA, Jones NS, Ouldridge TE, 2017, What we learn from the learning rate, J. Stat. Mech.
- Ouldridge TE, 2017, The importance of thermodynamics for molecular systems, and the importance of molecular systems for thermodynamics, Nat. Comput.
- Ouldridge TE, ten Wolde PR, 2017, Fundamental costs in the production and destruction of persistent polymer copies, Phys. Rev. Lett.
- Ouldridge TE, Govern CC, ten Wolde PR, 2017, Thermodynamics of computational copying in biochemical system, Phys. Rev. X.
- McGrath T, Jones NS, ten Wolde PR, Ouldridge TE, 2017, Biochemical machines for the interconversion of mutual information and work, Phys. Rev. Lett.
- ten Wolde PR, Becker NB, Ouldridge TE, Mugler A, 2015, Fundamental Limits to Cellular Sensing, J. Stat. Phys.
- Ouldridge TE, ten Wolde PR, 2014, The robustness of proofreading to crowding-induced pseudo-processivity in the MAPK pathway, Biophysical Journal.
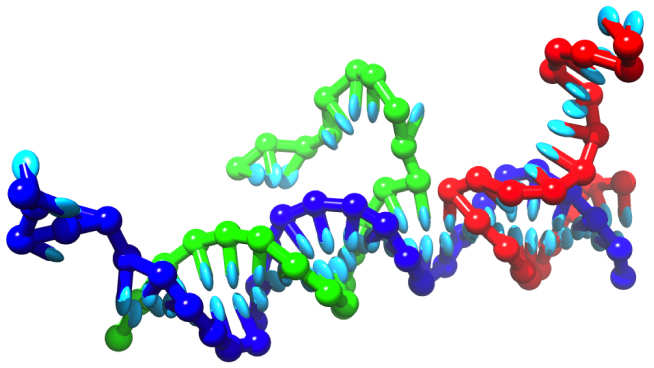
The elegant selectivity of Watson-Crick base-pairing makes DNA an extremely useful tool for the construction of nanoscale objects and machines. Stable structures and mechanical cycles can be programmed into a system of single strands by careful choice of the sequences of bases. I'm particularly interested in using nucleic acids to design artificial analogs of complex cellular systems, to enable careful exploration of the design principles and engineering possibilities.
Despite the experimental successes, there is no clear theoretical description of the processes involved. We have developed a nucleotide-level coarse grained model of DNA, oxDNA, which is detailed enough to capture the essential physics of assembly processes, yet simple enough to be applicable over long time scales. Code, user guides and examples for simulating the model can be downloaded from this site.
The oxDNA model was developed in the Doye / Louis groups in Oxford. It has since been applied in collaboration with the Turberfield group in Oxford, the Winfree group in Caltech and the Nir group at the Ben-Gurion University of Negev, as well as being used independently by other researchers.
Even with oxDNA, it is still not practical to simulate the formation of very large structures. A collaboration with the Turberfield and Kwiatkowska groups in Oxford has led to a less detailed model that can describe the formation of DNA origami structures.
Relevant Publications
- Sengar A, Ouldridge TE, Henrich O, Rovigatti L, Sulc P, 2021, "A Primer on the oxDNA Model of DNA: When to Use it, How to Simulate it and How to Interpret the Results", Frontiers in Molecular Biosciences.
- Irmisch P, Ouldridge TE, Seidel R, 2020, "Modelling DNA-strand displacement reactions in the presence of base-pair mismatches", J. Am. Chem. Soc.
- Haley NEC, Ouldridge TE, Mullor Ruiz I, Geraldini A, Louis AA, Bath J and Turberfield AA, 2020, Design of hidden thermodynamic driving for non-equilibrium systems via mismatch elimination during DNA strand displacement, Nat. Comms.
- Fonseca P, Romano F, Schreck JS, Ouldridge TE, Doye JPK and Louis AA, 2018, Multi-scale coarse-graining for the study of assembly pathways in DNA-brick self assembly, J. Chem. Phys.
- Khara DC, Schreck JS, Tomov TE, Berger Y, Ouldridge TE, Doye JPK and Nir E, 2018, DNA bipedal motor walking dynamics: an experimental and theoretical study of the dependency on step size, Nucl. Acids Res.
- Snodin BEK, Romano F, Rovigatti L, Ouldridge TE, Louis AA, and Doye JPK, 2016, Direct Simulation of the Self-Assembly of a Small DNA Origami, ACS Nano.
- Dunn KE, Dannenberg F, Ouldridge TE, Kwiatkowska M, Turberfield AJ, Bath J, 2015, Guiding the folding pathway of DNA origami, Nature.
- Dannenberg F, Dunn KE, Bath J, Kwiatkowska M, Turberfield AJ, Ouldridge TE, 2015, Modelling DNA Origami Self-Assembly at the Domain Level, J. Chem. Phys.
- Snodin BEK, Randisi F, Mosayebi M, Sulc P, Schreck JS, Romano F, Ouldridge TE, Tsukanov R, Nir E, Louis AA, Doye JPK, 2015, Introducing improved structural properties and salt dependence into a coarse-grained model of DNA, J. Chem. Phys.
- Schreck JS, Ouldridge TE, Romano F, Sulc P, Shaw L, Louis AA, Doye JPK, 2015, DNA hairpins primarily promote duplex melting rather than inhibiting hybridization, Nucleic Acids Research.
- Mosayebi M, Louis AA, Doye JPK, Ouldridge TE, 2015, Force-induced rupture of a DNA duplex, ACS Nano.
- Machinek RR, Ouldridge TE, Haley NE, Bath J, Turberfield AJ, 2014, Programmable energy landscapes for kinetic control of DNA strand displacement, Nature Communications.
- Doye JPK, Ouldridge TE, Louis AA, Romano F, Sulc P, Matek C, Snodin BEK, Rovigatti L, Schreck JS, Harrison RM, Smith WPJ, 2013, Coarse-graining DNA for simulations of DNA nanotechnology, Physical Chemistry Chemical Physics.
- Srinivas N, Ouldridge TE, Sulc P, Schaeffer JM, Yurke B, Louis AA, Doye JPK, Winfree E, 2013, On the biophysics and kinetics of toehold-mediated DNA strand displacement, Nucleic Acids Research.
- Ouldridge TE, Sulc P, Romano F, Doye JPK, Louis AA, 2013, DNA hybridization kinetics: Zippering, internal displacement and sequence dependence, Nucleic Acids Research.
- Ouldridge TE, Hoare RL, Louis AA, Doye JPK, Bath J, Turberfield AJ, 2013, Optimizing DNA nanotechnology through coarse-grained modeling: A two-footed DNA walker, ACS Nano.
- Sulc P, Romano F, Ouldridge TE, Rovigatti L, Doye JPK, Louis AA, 2012, Sequence-dependent thermodynamics of a coarse-grained DNA model, Journal of Chemical Physics.
- Ouldridge TE, Louis AA, Doye JPK, 2011, Structural, mechanical, and thermodynamic properties of a coarse-grained DNA model, Journal of Chemical Physics.
- Ouldridge TE, Louis AA, Doye JPK, 2010, DNA nanotweezers studied with a coarse-grained model of DNA, Physical Review Letters.
Thermodynamics, the science of heat and energy transfer, emerged as a field in the 19th century, motivated by the need to describe the engines that powered the industrial revolution. One of the challenges of modern science is to adapt and extend the theory to describe microscopic systems in which fluctuations play a key role. Biological and biologically-inspired systems are a key arena for these new ideas, due both to the need to understand natural molecular analogues of the engines and processes that we are familiar with at much larger length scales, and the possibility of developing artificial devices ourselves.
Not only does thermodynamics provide understanding of biological systems, but the study of real biophysical devices in turn provides us with a deeper understanding of the thermodynamic principles at play. In particular, the natural diffusive behaviour of biomolecules allows us to study complex systems that do not require external manipulation to function.
Relevant Publications
- Ouldridge TE and Wolpert DH, 2023. Thermodynamics of deterministic finite automata operating locally and periodically. New Journal of Physics.
-
Seet I, Ouldridge TE, Doye JPK, 2023, Simulation of reversible molecular mechanical logic gates and circuits, Physical Review E.
- Qureshi B, Juritz J, Poulton JM, Beersing-Vasquez A, Ouldridge, TE, 2023, A universal method for analyzing copolymer growth. The Journal of Chemical Physics.
- Juritz J, Poulton JM, Ouldridge TE, 2021, "Minimal mechanism for cyclic templating of length-controlled copolymers under isothermal conditions", The Journal of Chemical Physics.
- Poulton JM, Ouldridge TE, 2021, "Edge-effects dominate copying thermodynamics for finite-length molecular oligomers", New J. Phys.
- Ouldridge TE, 2020, A biochemical device to demystify a century-old thermodynamics puzzle from theoretical physics, Reseach Outreach.
- Brittain RA, Jones NS, Ouldridge TE, 2019, Biochemical Szilard engines for memory-limited inference, New. J. Phys.
- Ouldridge TW, Brittain RA, ten Wolde PR, 2019, The power of being explicit: demystifying work, heat, and free energy in the physics of computation, in "The Energetics of Computing in Life and Machines", SFI Press.
- Stopnitzky E, Still S, Ouldridge TE and Altenberg L, 2019, Physical Limitations of Work Extraction from Temporal Correlations, Phys. Rev. E.
- Poulton J, ten Wolde PR and Ouldridge TE, 2019, Non-equilibrium correlations in minimal dynamical models of polymer copying, PNAS.
- Deshpande A, Ouldridge TE, 2017, High rates of fuel consumption are not required by insulating motifs to suppress retroactivity in biochemical circuits, Engineering Biology: 1.
- Deshpande A, Gopalkrishnan M, Ouldridge TE, Jones NS, 2017, Designing the Optimal Bit: Balancing Energetic Cost, Speed and Reliability, Proc. Roy. Soc. A.
- Brittain RA, Jones NS, Ouldridge TE, 2017, What we learn from the learning rate, J. Stat. Mech.
- Ouldridge TE, 2017, The importance of thermodynamics for molecular systems, and the importance of molecular systems for thermodynamics, Nat. Comput.
- Ouldridge TE, ten Wolde PR, 2017, Fundamental costs in the production and destruction of persistent polymer copies, Phys. Rev. Lett.
- Ouldridge TE, Govern CC, ten Wolde PR, 2017, Thermodynamics of computational copying in biochemical system, Phys. Rev.
- McGrath T, Jones NS, ten Wolde PR, Ouldridge TE, 2017, Biochemical machines for the interconversion of mutual information and work, Phys. Rev. Lett.
Our work often involves systems that are too complex to be treated analytically. This means that simulations are a key tool in our research, and we are interested in simulation techniques and analysis tools for systems involving biomolecular reactions.
In this work we collaborate with the Doye / Louis groups in Oxford, the biochemical networks group of Pieter Rein ten Wolde in Amsterdam, Michael Tretyakov in Nottingham and Ruslan Davidchack in Leicester, and Oliver Henrich in Strathclyde.
Relevant Publications
- Qureshi B, Juritz J, Poulton JM, Beersing-Vasquez A, & Ouldridge TE, 2023, A universal method for analyzing copolymer growth. The Journal of Chemical Physics.
- Sengar A, Ouldridge TE, Henrich O, Rovigatti L, Sulc P, 2021, "A Primer on the oxDNA Model of DNA: When to Use it, How to Simulate it and How to Interpret the Results", Frontiers in Molecular Biosciences.
- Henrich O, Yair AGF, Curk T, Ouldridge TE, 2018, Coarse-grained simulation of DNA using LAMMPS, Eur. Phys. J. E.
- Davidchack RL, Ouldridge TE, Tretyakov MV, 2017, Geometric integrator for Langevin systems with quaternion-based rotational degrees of freedom and hydrodynamic interactions, J. Chem. Phys.
- Vijaykumar A, Ouldridge TE, ten Wolde PR, Bolhuis PG, 2017, Multiscale simulations of anisotropic particles combining Brownian Dynamics and Green's Function Reaction Dynamics, Journal of Chemical Physics.
- Davidchack RL, Ouldridge TE, Tretyakov MV, 2015, New Langevin and Gradient Thermostats for Rigid Body Dynamics, Journal of Chemical Physics.
- Ouldridge TE, 2012, Inferring bulk self-assembly properties from simulations of small systems with multiple constituent species and small systems in the grand canonical ensemble, Journal of Chemical Physics.
- Ouldridge TE, Louis AA, Doye JPK, 2010, Extracting bulk properties of self-assembling systems from small simulations, Journal of Physics: Condensed Matter.
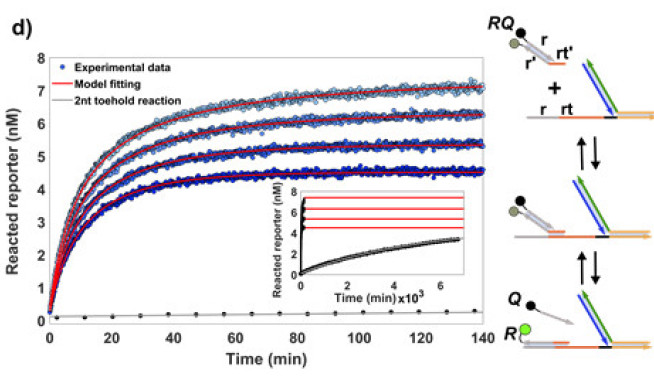
We've recently acquired our own (small) lab within the synthetic biology space at Imperial. We're using this lab to actually put our theoretical ideas into practice, engineering functional molecular systems from nucleic acids. These systems are both useful test-beds for our theory and engineering platforms for synthetic biology.
Our work currently focusses on engineering non-equilibrium information processing systems, analogs of the signalling and transcription/translation machinery in cells. To get a taste, check out Javi's talk at FNANO2020, or a simplified discussion of his overall project.
Below we list papers that present data obtained in our lab.
Relevant Publications
- Catala AC, Ouldridge TE, Stan GBV, Bae W, 2022, "Building an RNA-Based Toggle Switch Using Inhibitory RNA Aptamers", ACS Synthetic Biology,
- Bae W, Stan GBV, Ouldridge TE, 2021, "In situ generation of RNA complexes for synthetic molecular strand displacement circuits in autonomous systems", Nano Lett.
- Cabello-Garcia J, Bae W, Stan GBV, Ouldridge TE, 2021, "Handhold-mediated strand displacement: a nucleic acid-based mechanism for generating far-from-equilibrium assemblies through templated reactions", ACS Nano.
- Haley NEC, Ouldridge TE, Mullor Ruiz I, Geraldini A, Louis AA, Bath J and Turberfield AA, 2020, Design of hidden thermodynamic driving for non-equilibrium systems via mismatch elimination during DNA strand displacement, Nat. Comms.