Gatemons
Superconducting circuits are a leading solid-state platform used by academic teams, publicly funded national initiatives, and international technology companies for developing quantum computers. At the heart of these circuits are Josephson junctions (JJs), superconducting layers separated by a thin non-superconducting spacer. JJs introduce a nonlinear relation between currents and voltages, quantified through the Josephson inductance (LJ). For quantum computing applications, LJ is the key to producing a ladder of uniquely addressable microwave transitions, the lowest of which can form the logical states of a quantum bit – or “qubit” [Krantz2019]. Conventional circuits employ JJs with oxide spacers, whose LJ is fixed by the JJ dimensions. Tuning the nonlinearity and qubit transition frequency, a key operation required for applications, is achieved by fabricating two oxide JJs in a superconducting quantum interference device (SQUID) and modulating LJ using the magnetic field generated by local currents. The associated crosstalk and heat dissipation is a major obstacle to building large arrays required for practical quantum computing and simulation. There have been many innovations in circuit design over the last two decades, but the oxide JJ has remained largely unchanged. The need for innovation has revived interest in replacing the JJ by a Josephson Field-Effect Transistor (Jo-FET), a semiconductor channel whose LJ is controlled by capacitively coupled gates rather than currents. Electrons within the semiconductor channel form bound states between the two superconductors, and a local gate voltage changes the bound state energy, tuning the transition frequency with negligible crosstalk and heat. These gate-tuneable transmons – or gatemons –, have been realised with a range of Jo-FETs, but their lifetimes and reproducibility are not competitive with existing oxide technology [Aguado2020]. We are working on the breakthroughs required to ensure gatemons reach their full technological and commercial potential.
Superconducting Spin Qubits
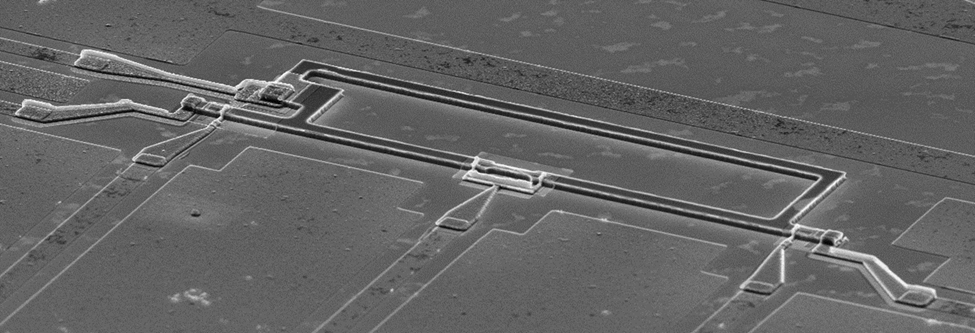
Exploiting the spin angular momentum of single electrons confined in semiconductors is at the forefront of efforts to realise scalable high-quality qubits for solid-state quantum computing. Unlike superconducting qubits, which have modest lifetimes and are easy to fabricate in large numbers, spins have longer coherence times but are harder to manipulate and entangle. Recent results demonstrate a promising hybrid platform that combines the best of both these technologies: a superconducting spin qubit. The spin belongs to an electron trapped in a semiconductor channel as it bounces back and forth between the superconducting contacts of a Josephson junction. The main novelty is that the quantum state of the spin couples directly to the Josephson supercurrent, a macroscopic degree of freedom that can be easily detected and manipulated using scalable microwave circuits to couple remote spins.