How animal research is helping fight antibiotic resistance

We explore how animal research is playing a vital role in the battle against antibiotic resistant superbugs.
People do not expect to die from a simple infection. But that might change: the world is running out of effective antibiotics.
For decades, diseases like bacterial gastroenteritis and colitis have not been a serious health threat, thanks to antibiotics. These small molecules are able to kill bacteria or stop them from reproducing and spreading in the body. However, before antibiotics became available, the overall mortality for bacterial infection was more than 70%. Since the discovery of penicillin, the range of antibiotics used to treat infections has increased enormously.
"The way bacteria are killed by antibiotics in lab cultures is very different from the way they respond to the same drugs in an animal." - Dr Sophie Helaine
Unfortunately, the bacteria causing diseases have evolved too, and the excessive or inappropriate use of antibiotics has led to the emergence of resistant strains, which do not respond any more to antibiotic treatment. The adaptive capacity of bacteria, a natural process observed since the first antibiotic agent was discovered, has increasingly become a problem.
Without effective antibiotics for the prevention and treatment of infections, bacteria persist in the body, increasing the risk of spread to others. Medical procedures such as organ transplantation, cancer chemotherapy, diabetes management and major surgery (for example, caesarean sections or hip replacements) become very high risk and doctors lack new drugs to challenge these emerging superbugs.
In the search for new antibiotics or a new way to fight the superbugs, research involving animals plays a key role. Anna Napolitano spoke to two Imperial researchers in the field about their work, and why it is still necessary to use animals.
Bacteria are fighting back
Dr Sophie Helaine, former senior lecturer of the CMBIs at Imperial College and now at the Harvard School of Medicine, has focused on finding ways to defeat superbugs since the early days of her career.
Sophie hopes to find a vaccine against Salmonella infection, a commonly spread bacteria responsible for a wide range of diseases, from gastroenteritis to typhoid fever. Once easy to control with antibiotic treatments, nowadays multidrug-resistant bacterial strains have become a difficult enemy to fight.
For her studies, Sophie uses mice, as they are a natural host for some Salmonella strains. Mouse models of Salmonella have been used for a long time, and scientists have learned lots of useful information from them about these terrible bacteria.
“Up to now antibiotics were screened in cells, and the one that was working in cells were then tested in animals and finally in clinical trials,” she said. “However, the way bacteria are killed by antibiotics in lab cultures is very different from the way they respond to the same drugs in an animal.
“Scientists worldwide have realised that lots of compounds that looked unable to kill bacteria are actually excellent when the bacteria is already struggling to fight the immune response of the infected organism. This clearly demonstrated the importance of testing compounds during a real infection.”
Dr Helaine works primarily on understanding the basis of the infection: how bacteria establish an infection once they are in the organism, and which signals it uses to start the infectious state to its own advantage. Knowing more about this initial process is essential to understand why the existent antibiotics sometimes stop working and finding new ways to optimise them.
“There was no way we could use genetically modified bacteria in patients, and without them we would not be able to really understand the molecular principle of the infection and why some bacteria, called persistent, are able to escape the antibiotic treatment and persist in the body as reserve that will give rise to new, more aggressive diseases”.
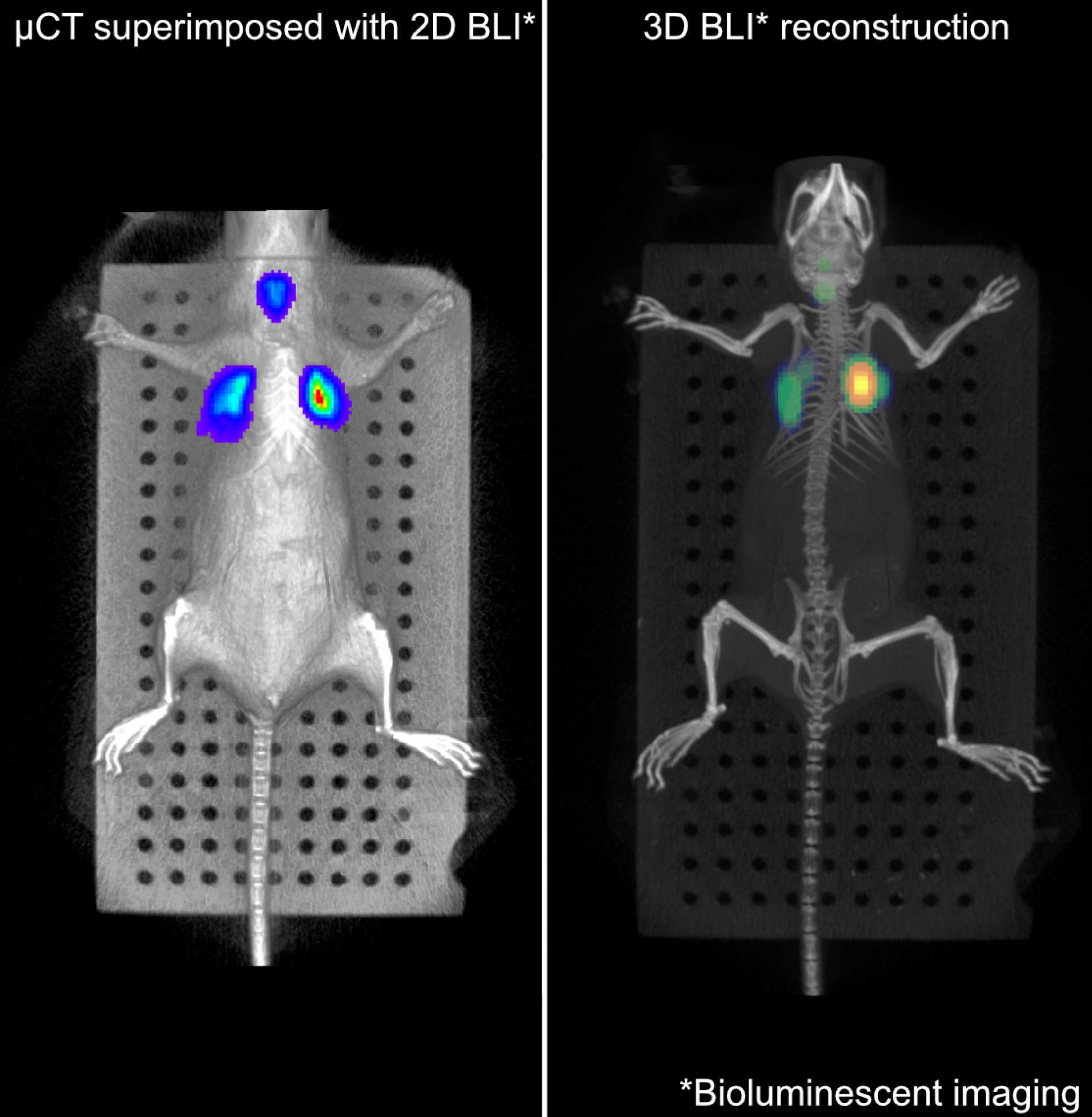
Use of animals does have some limitations. Laboratory animals are kept under specific conditions that differ from what happens in real life. On the one hand, this is useful because researchers can study a specific infection without so-called ‘disturbing factors’ (all the conditions that are presents in a wild environment, like other pathologies or factors, and that can influence the readout of an infection with a single organism). Using laboratory animals, they can also analyse all the effects that the specific disease has on the organs of the living body and dissect how the disease evolve from the initial infection to the final stages.
However, a patient’s response to infection can be much more complicated than what happen in a laboratory animal, and multiple factors can influence the outcome of the disease. For this reason, animal testing is always seen as stepping stone toward new treatments.
According to Dr Helaine, this hurdle is nevertheless essential: going from test tube to human can be very dangerous without all the information gained through the intermediate studies in animals. Moreover, there is much more than we can learn from animal studies: how exactly the drugs works, how the immune system behaves in the presence of the infection, and so on. The study of all of those factors directly in humans is not feasible.
Understanding the microbiome
Professor Gad Frankel, of the Faculty of Natural Sciences, also uses animal models in his research. His work focuses mainly on gut and lung infections, caused by two different bacteria: Escherichia coli and Klebsiella pneumonia. Professor Frankel believes that the mice models he uses are essential to fight the emergence of antibiotic resistant strains.
“A lot of stuff can be done in Petri dishes, but then when it comes to testing the efficacy and potency of antibiotics, one has to look at animals models.”
The microbiome will continue to play a role and to understand what this role is, we need to use animals Professor Gad Frankel Faculty of Natural Sciences
As Professor Frankel explains, antibiotics are mainly given orally, and, usually, they are not selective: antibiotic treatment not only kills the dangerous bacteria but also all the commensal bacteria that live in our bodies and are essential for our wellbeing – the so-called microbiota.
“Is essential to understand what the impact of antibiotics on microbiota is, and how this impacts on the spreading of the disease. We also need to understand how to mitigate the negative effects and help the gut microbiota to recovery from the shock of antibiotic treatment.”
For now, using animal models is by far the most effective way of understanding how antibiotics work in an organism, Professor Frankel explains. Some research uses organoids - three-dimensional tissue cultures that replicate much of the complexity of an organ - but these have limitations. Professor Frankel says:
“Organoids give you a lot of information, but do they accurately and completely recapitulate what happens in the body? If you look at gut organoids, for example, they do not usually have gut microbiota present or the immune system around. You need to recreate it, and it is not going to be as complex as what you find in the gut of a living organism”.
These cell culture systems are beneficial for studying how infections develop. However, as Professor Frankel says: “For infection, I think, there is something still missing in the lab system. And it doesn’t really matter if it is lung infection or a gut infection, the microbiome will continue to play a role and to understand what this role is, we need to use animals”.
Overcoming resistance
For patients that arrive at the hospital severely unwell with infection, doctors have to make a near instant decision about the type of antibiotic to give – there is no time to profile the bacteria infecting or check if they are resistant to antibiotics. Sometimes the decision is wrong, and patients receive an antibiotic for which the bacteria causing the disease are resistant. An important question is if this inadvertent misuse of the antibiotic is neutral or can worsen the situation.
Professor Frankel is using animal models to investigate this. Despite the substantial differences between mice and humans, if we are able to replicate the clinical situation in the mouse model, then the results can be translated to patient care. In his research, Professor Frankel models what happens during lung infections with resistant Klebsiella when they are treated with antibiotics to which the bacteria is resistant.
“I have a wonderful clinical fellow (Dr Josh Wong), he is a medical doctor, and he came to me about two years ago. He works in intensive care units, and he said to me – I’m losing many of my patients to Klebsiella infection as they are antibiotic resistant, I want to understand what is going on. It was an opportunity to come closer to the front line of the infection.”
Whilst it is necessary to use animals for this important work, the researchers limit the numbers of animals they have to use.
As Professor Frankel points out, “In our animal facility, we have probably one of the most sophisticated in vivo imaging platforms available, so we can look at colonisation in real time using bioluminescent bacteria. This allows us to follow the same animal over time with better monitoring of the development of the disease, whilst using fewer animals.
“Moreover, we have purchased a very sophisticated monitoring system which in the future will allow us to measure physiological parameters (heart rate, respiration rate and oxygen saturation) continuously, so that we will be able to switch from having time post-infection endpoints, to having clinical endpoints: a system that resembles what happens to humans. Having such physiological measurements will also reduce experimental variability, again allowing us to use fewer animals to have meaningful and robust results”.
Thanks to those technologies, Dr Wong and Professor Frankel will be able to find new targets to develop new antibiotics or new treatments against resistant bacteria, using fewer mice.
Article text (excluding photos or graphics) © Imperial College London.
Photos and graphics subject to third party copyright used with permission or © Imperial College London.